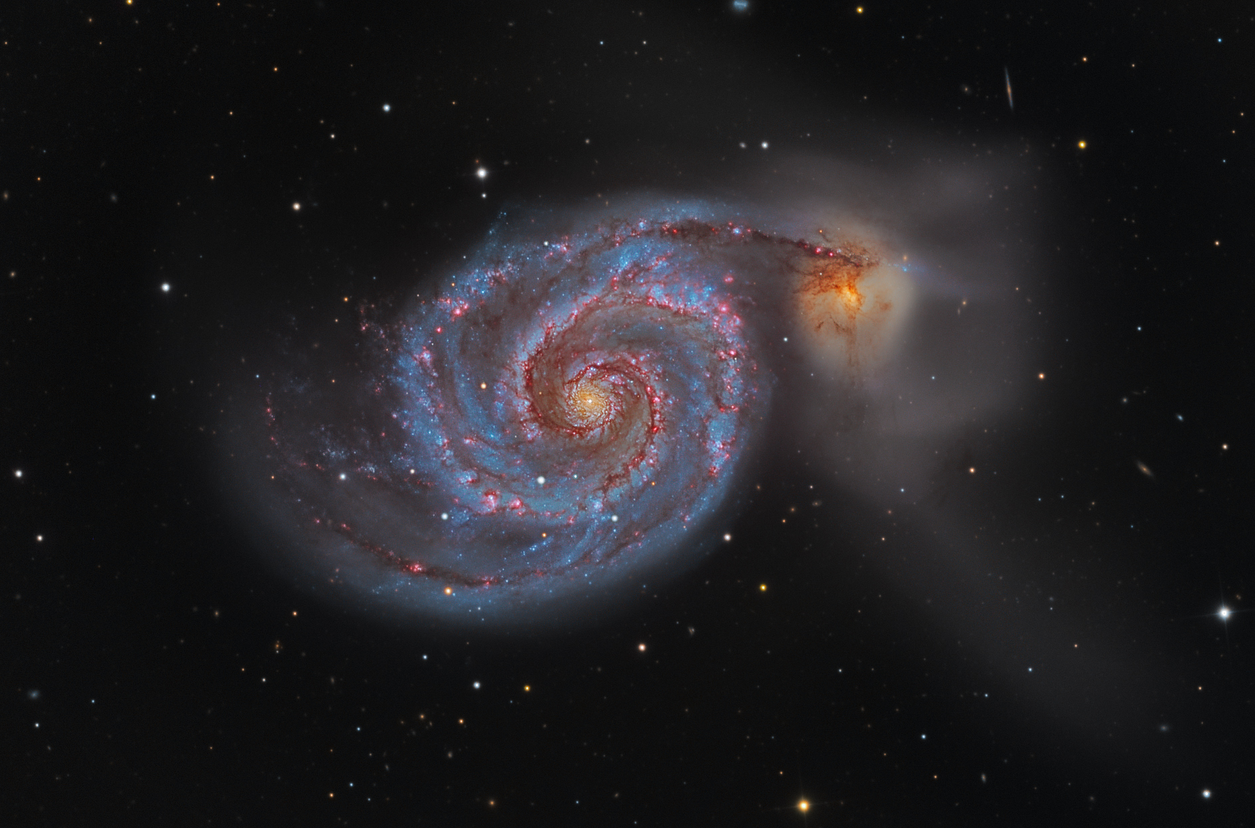
Basics of Astronomy
These are my notes and worked examples on the Basics of Astronomy.
Table of Contents
- Astronomy Essentials For Beginners
- How Do Telescopes Work
- Important Stuff In The Galaxy
- Orbital Motion
- Why Does The Earth Support Life
- The Moon
- The Cratered World Of Mercury
- The Rocky Planet Venus
- Mars, The Red Planet
- The Solar System And Beyond
- Why Is There Debris In The Galaxy
- Radiation
- Spectroscopy
- Exoplanets
- How The Sun Works
- Stars
- How Stars Form
- How Stars Evolve
- Exploding Stars
- Matter in Interstellar Space
- Black Holes
- Galaxies
- Classification of Galaxies
- Dark Matter
Astronomy Essentials For Beginners
First, who doesn’t just love Astronomy? Okay, I am sure there are some before people try to correct me. However, if you’re here, I am just going to assume that you have at least some passing interest if you are here and reading this.
I love Astronomy. It started by looking at those fascinating pictures of galaxies that get posted all the time on the internet when I was a kid. Those big composites with their color fixing did it for me.
Ever since then I have been interested. From there, I developed several other interests that were offshoots of Astronomy such as Mathematics, Physics, Programming, and yes even Writing.
Beginnings of Astronomy
We need to look at some of the history of Astronomy. Now, I’m not trying to write a book here so I will have to gloss over a few things. I want to get the highlights, though, so everything makes some sense for you.
We will assume the first interest in Astronomy came from the first primitive people in the world. I can’t even imagine what they thought when they looked up at the sky for the first time.
I am sure there was interest, curiosity, and maybe even dread at the unknown. This was the state of astronomy for many thousands of years. Not much they could do about it.
The Telescope

The first advancement was the creation of the telescope. It had been a long time coming. Glass and mirrors had been manufactured for quite a while before someone thought to apply it to look at the heavens.
Galileo is who we recognize today as the first to make good strides in using the telescope. He didn’t invent it, nor was he the first to use one. What he was good at, though, was in optimizing and improving what was already out there.
He had refracting telescopes and was very good at refining what they did. For the first time, people could see the heavens in better detail. Planets were visible now, and they were not just a dot in the night sky.
Galileo could see other objects, but at first he did not know what he was looking at. This was in the early 1600s.
Kepler made the next large advancements by using a convex lens. A convex lens magnified celestial objects. This led to many discoveries.
Motion of Objects
One of the most influential discoveries was explaining how objects in space moved. These were his laws of planetary motion and the basis of celestial mechanics that we use today.
These laws relied on the concept of heliocentrism, which was very advanced for the time. Heliocentrism basically meant that the Sun was the major force in the solar system.
Gravity
The concept of gravity is the next advancement that spurred things along. Gravity was also independently worked on by multiple people.
This was a good indicator that they knew how important it was going to be. Gravity, if powerful enough, influences everything.
It won’t have a noticeable effect on small objects. However, large objects in space all affect each other. Of course, the Sun is the dominant force in the solar system.
The planets orbit around the Sun because of gravity. At some point, the different planets were each captured by the Sun and it would take some significant force to knock them out of the reach of its gravity.
Light
Once we got an understanding of gravity, the light that we saw in space started getting attention. Astronomers started studying it. One of the first realizations was how fast it had to be.
Many experiments were done. At first these experiments were just confusing because our system of measurements and scientific equipment could not accurately portray light’s speed.
Eventually the early astronomers got a handle on it. One of the most important discoveries made was that speed is a constant. Its speed is always the same.
This is important because it allows us to measure distances to other things in space. By doing this, we can tell how far away something is and if that object in question is moving.
Astronomers got so excited about the study of light that a new field was created. This field is spectroscopy.
Spectroscopy
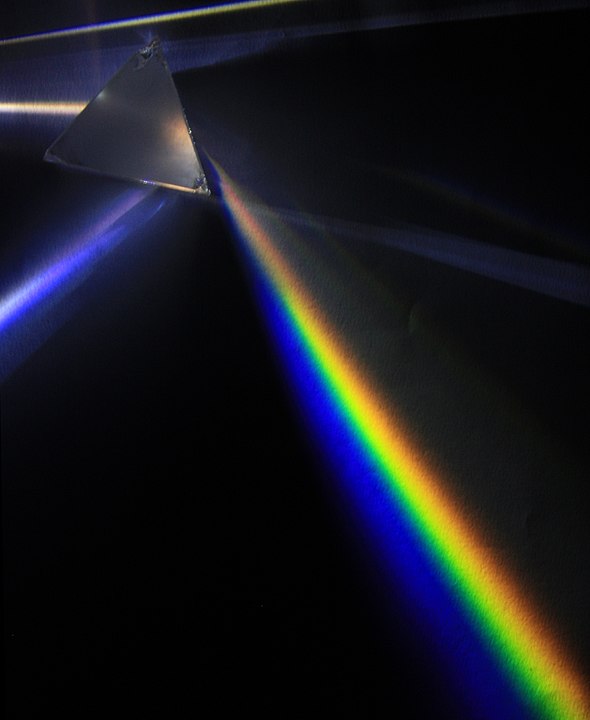
Light is radiation. Visible and non-visible light is all just radiation. This is interesting because most objects in space emit multiple forms of radiation.
Scientists study them as much as possible because they give us clues about what is going on.
Studying the light from stars, for example, can tell us relative distances and whether a star is moving. There are many other details light can tell us too, such as the temperature and what it is made of.
Classifying Stars
One of the first things Astronomers figured out a long time ago was that almost every star is different. They were of different ages, had different temperatures, and rotated at different speeds.
The next task was classifying all these different stars with similar characteristics. This has been a large and ongoing job, as you can imagine.
Eventually arbitrary classes were created so that we could better group stars. There are several groups and they all mean different things.
Life Cycles of Stars
Stars come from molecular clouds. Molecular clouds are part of nebulae. These enormous clouds of dust can form into a star. This happens by gravity usually but there have been other ways occasionally.
The gravity of a close celestial object can grab and condense a molecular cloud. After it is condensed enough, processes begin the long journey to becoming a star.
The Solar System
At this point we have a good idea what makes up our solar system. People have been constantly mapping it out since telescopes were invented. So this is a process that has been going on for hundreds of years.
It is called the solar system because that is the area that our Sun is dominant over.
Space objects include:
- comets
- asteroids
- planets
- moons
- stars
- galaxies
- nebulae
- dust clouds
- black holes
All of these are very different and have different origin stories.
One way we know their differences is because we compare everything once we measure everything. It is very insightful.
There is obviously a lot more to the solar system than I can mention here. Certainly each topic is a book itself. I will say that most people get really curious about planets at this point.
Are there planets that we have missed out there? Are there any planets that are exactly like Earth? That alone is an acceptable topic to explore.
Space Debris
If you study different objects, you quickly see that debris makes up a large part of our galaxy. They include asteroids and comets in this group. When celestial size objects collide, stuff flies everywhere.
This is where asteroids, comets, and large clumps of dust come from. How they all interact with each other is just fascinating!
Some asteroids are huge and every so often travel pretty close to Earth. This always makes for big news so watch for these events in your favorite publication.
Comets are fun too. When these get close I highly recommend grabbing your telescope or a good pair of binoculars. These are amazing to see and even better done with friends. These parties are great.
You may not think about it, but dust clouds play big roles in the galaxy. It is often the formational material for stars and planets. It’s the glue, so to speak.
Vast clouds can also blot out the sky in certain areas. A gigantic cloud can just absorb all the light and make portions of space look like nothing is there.
Star Properties
Stars are wonderful objects. Our own Sun is the closest star to Earth. Studies of it and others are very involved. They each have their own:
- size
- orbital velocity
- rotation
- temperature
- brightness
Different temperatures usually imply a relative age. This is because stars usually have an evolution to follow. Different stages usually involve certain temperature ranges.
The size of stars is also very interesting. It is interesting because often that can tell us how it formed. Once we know how a star formed we can infer many other facts about it. Is it in a binary system?
Is it still growing through an accretion disk? Any question we can successfully answer leads to even more knowledge and questions to explore. It can be a deep rabbit hole indeed, but it is so very fun once you get started.
Star Formation
So now you may wonder how stars formed. That is an excellent question and is another area of study in Astronomy.
To put it as short as possible, stars form in the middle of huge gas and dust clouds when the cloud’s own gravity becomes very strong.
Much of the gas and dust is condensed and they generate so much heat that nuclear fusion happens. There is an immense amount of pressure in the middle of new construct and we can now call this a protostar. I have skipped a lot, but that should give you an idea.
Shockwaves are often the start of star formation. They often form right in the middle of the now compressed cloud. They then travel through the cloud in many directions at once.
This action will further compress and heat the material inside. This is so catastrophic to the cloud that it will separate into distinct parts. These distinct parts will now usually become stars themselves.
Star Explosions
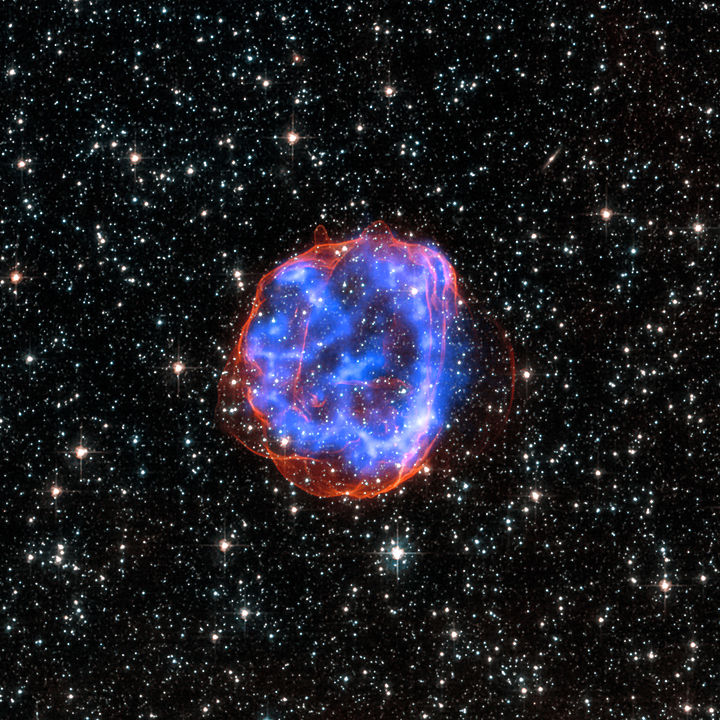
We have just mentioned how stars are formed. What happens when they die? This is another superb topic of study that astronomers have to choose from. When stars explode, we can see nova and the awesome supernovae.
These explosions often involve a binary system. The reason is that one star takes material from the other star. These have been called vampire stars for this reason.
While this vampire star is stealing energy, it gets super hot and dense. Anyway, after a while, one star gets too much material to handle. The pressure has also increased.
The star can’t take anymore. Its core has been squeezed by gravity until it is super tight and dense. Think of our planet squeezed so small it’s like the size of a golf ball.
I’m sure that analogy isn’t exactly right but just pretend with me. So, our golf ball can’t stay like that forever. The force compressing it will eventually give way.
What happens next? Well, an explosion happens next. It is an explosion so violent and large it makes anything that happens on Earth look like a balloon popping in comparison. That is what we know as a supernova!
Neutron Stars
Some supernovae turn into neutron stars. This is a misleading term. It’s not really a star anymore at this point. It is basically a ball of neutrons that defy the laws of physics as we know it.
So, after the explosion has finished, which can take a very long time, all the star’s material will get blasted away. The only thing left will be the core of the previous star.
We call this a supernovae remnant. It can take up the space of a small county here on Earth but have the mass of our Sun for example. This density is beyond comprehension.
Neutron stars can also spin. Some spin hundreds of times a second. Think about what has to be going on in the inside for something with the mass of our Sun to spin hundreds of times per second.
Truly incredible in my opinion. Neutron stars that exhibit this behavior are called pulsars. I’m sure you might have heard of them.
Magnetars
Astronomers are still trying to discover more about magnetars. There have been very few discovered. Astronomers have also had a hard time catching them in action.
Their bursts are very sporadic. It has a magnetic field around a thousand times greater than a typical neutron star.
They are about the same size and mass as a neutron star but the difference is the magnetic field. Many stars have only a slight magnetic field.
So we do not know why some of these stars, namely magnetars, have magnetic fields thousand and millions of times stronger. It is surely an interesting area of research.
Magnetars are also a source of x-ray and gamma-ray bursts. These bursts are formed by starquakes on the surface which disturb the magnetic field. There are some theories about why the magnetic field is so strong but I will say that I don’t understand them yet.
They involve super dense fluids after a neutron star forms. I need to read up on them more but there is also very little literature to read, which is the problem.
Black Holes
We do not know a lot about black holes. For the longest, they were just theoretical. Now we have more evidence about them, but not much. They are really just a super dense neutron star with gravity so strong that it sucks everything into them, even light.
Objects would never disappear into a black hole. The gravity is too strong, it would disintegrate the object into atoms before it even got close.
There are many what-if scenarios that scientists have conjectured. Not much has really turned out to be true yet as getting any information from a black hole is incredibly difficult.
The only exception to that is Astronomers think a black hole can’t be larger than 3 solar masses, relative to our own Sun.
Conclusion
In this article, we have discussed how you learn about Astronomy. I have summarized several important topics. Each one is worthy of study. Nothing is more important than the other.
I did have to leave several things out, but I tried to get most of the important ideas.
In case you are wondering, my favorite areas of study are the fast pulsars and magnetars. We know so little, so I am sure there will be momentous discoveries in this area one day.
How Do Telescopes Work
Telescopes are being used differently as time goes on. We used to only use them to see objects in normal light. Now we can leverage our technology gains to see different wavelengths of light in the universe.
This has opened up our studies with a lot more data than we previously had. This article talks about the different fields and categories of astronomy and what they mean.
Essentials of Telescopes
There are many kinds of telescopes. With many uses as well, there is a diverse market for every kind of telescope you could imagine. Narrowing down what your goal is can save you a lot of money in the end. When looking for a telescope, there are a few terms you should know.
Aperture - This is the size of the mirror and is the most important factor.
Focal Length - This is the distance from the mirror to the focal point.
Magnification - This depends on the focal length and is a good indicator of the telescope.
Focal Ratio - The focal ratio is the relationship between its focal length and the aperture of the telescope.
To do any meaningful research, you need a telescope, a wavelength filter or spectrometer, and a detector. The telescope needs to be as large as possible. A larger aperture gathers more light.
Bigger telescopes see fainter objects. Once they gather light, they can focus this light into an image. This is how they generally work, no matter the type of radiation.
The wavelength filter can be a variety of devices. Filters can be simple or complex. They can show you one color or a rainbow. It depends on what you are after. Different colors give different information.
After light has been collected, you must use a device to detect the wavelengths you are after. With a detector, you can get long exposures. This is key to observing faint objects far away.
Optical Telescopes
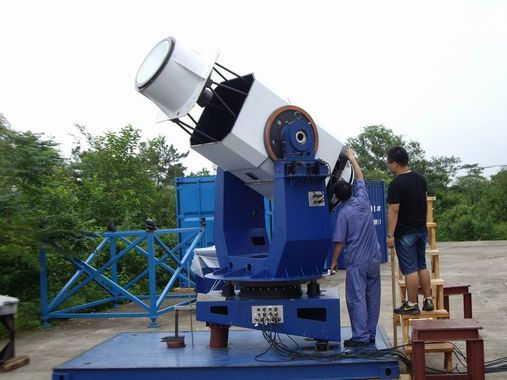
Optical telescopes collect visible light. That is why they are optical. Seems simple enough, right? It really is. The more light we then collect, the more we can see.
When people think of telescopes, these are the type that are thought of when first learning the basics of telescopes. It is how we use telescopes. There are two main kinds of optical telescopes:
Refracting- bends light that is then focused.
Reflecting- uses a mirror to reflect light.
Refracting scopes use a lens to gather and concentrate light. They do not do colors as accurately as they should either. A lens will also absorb some frequencies through the atmosphere. This makes a refracting telescope a poor choice for infrared studies.
Using a lens in your telescope is also more expensive. This is because a lot more quality and effort goes into a lens.
Reflecting telescopes use mirrors instead of a lens to work with the light that is captured. Since they use a mirror, there are no absorption issues to deal with. You can also use a much larger mirror than a lens.
So scopes that are large almost always use a mirror. Among reflecting telescopes there are different ones as well:
Newtonian-reflected to an eyepiece at side of instrument
Cassegrain-light reflects light at the end of the instrument
The Keck Telescope and the Hubble Space Telescope are the two most famous. They based Keck in Hawaii while Hubble is orbiting in space.
Size Of Telescopes
The size of telescopes has grown over time. Originally they were pretty small in Galileo’s day. However, thanks to modern science, we are getting larger ones all the time. Indeed, the space telescopes are also getting bigger.
What is the advantage to a larger telescope you may ask? Well, the larger the telescope the more light it can collect. It is all about the amount of light it can gather. This helps us see far off objects.
The telescope’s mirror directly affects how much we can see. This helps with another issue, which is the telescope’s resolving power. Its resolution is also very important because it lets us see the details of objects in space.
For example, it lets us study far off objects and refine our theory of star classification.
A larger mirror also helps with diffraction. This is the tendency of light to bend. Basically, the larger the mirror the less bending of light, or diffraction, that you will have.
Presently, telescopes can be huge. The largest ones are 10-11 meters in diameter. Larger ones are being built. I imagine, there will always be a larger one being built.
Imaging With Telescopes
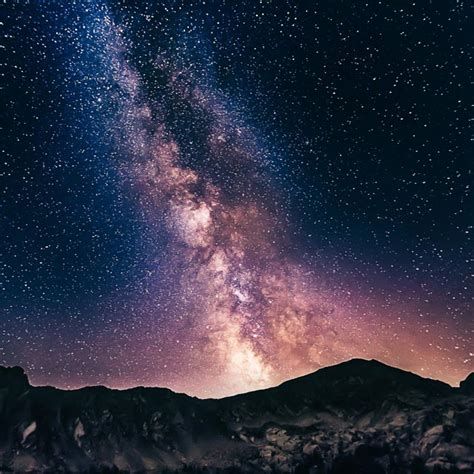
Imaging and the field of astrophotography are very popular these days because looking through a telescope is looking back in time. When individuals first want to know how telescopes are used, imaging the night sky is one of the first choices.
More and more people all the time are doing this because of how useful and fun it is. The pictures created are just breathtaking. Computers and associated software are being used to make this process even easier and more reliable.
Done correctly, they can even reduce background noise in pictures. The background noise also has its own characteristics. It can then be analyzed and useful information will be the result.
So what exactly is imaging then you may ask? It is the process of taking very long-exposure photographs of cosmic objects. While your camera is doing this it goes onto its film.
There is a slight problem with this though. Can you guess? Yep, the Earth and everything moves. For astrophotographers, to take those stunning pictures the telescope has to move.
If you are interested in astrophotography, then you will buy a telescope that has a guidance system included in it that will track planets and stars.
This means there is a small computer that will adjust position in far greater detail than we could ever do by hand. The purpose is to keep our object that is being photographed squared.
Most often this was done by hand, and it could be quite difficult. It was easy to make errors doing it this way.
CCD Chips
Fairly recently technology has advanced and new CCD chips have come on to the market. CCD stands for charged coupled devices. These chips take digital images of objects. On the CCD, a pixel stores the photonic information that it gathers.
Just like the previous method it takes time to gather enough light and information on to these chips but the results can be wonderful. These CCD’s record starlight and allow much greater visibility into the heavens.
This process allows us to detect and analyze the faintest objects. Without doing this, we would not know they were there.
These chips also can auto guide, making the following of an object a much simpler process if you have the cash to pay for the hardware.
These chips are much more efficient than previous methods. Think cell phone camera versus Polaroid.
This leads to the next subject, which can be very useful. It is the study of brightness of any object. We call this photometry. Astronomers combine photometry with the use of filters to analyze certain wavelengths.
As each range of wavelength can have unique characteristics, this is precious. To measure the light, a telescope takes it in and then we use an instrument called a photometer. It measures the amount of light received.
Spectroscopy, which is the study of the spectrum of light, goes right along with this. Without even looking at the source image, we can infer much from this and is one of the most important topics when dealing with the basics of telescopes.
It is hard to get splendid pictures while on Earth. It is not really our fault though. The atmosphere affects our view so much. Not a lot we can do from here. Therefore, we launched telescopes into space.
Turbulence in the air is constantly messing with our telescopes and imaging. An interesting fact is that turbulence has less effect on longer wavelengths.
I do not know why this is but guessing it is because smaller wavelengths are much more sensitive. Because of turbulence, we usually place telescopes at high altitudes.
Telescope Resolution
Resolution is the detail in an image. We want as much as possible. With higher resolution, the smallest features become apparent. This is vital in the study of astronomy. Aperture size is the key ingredient of resolution.
The larger the aperture, the sharper the image becomes.
Our atmosphere contains many gas pockets. These pockets catch and distort the starlight. They distort it by making it reach Earth in weird ways. This is the big problem with optical telescopes.
The distortion of our atmosphere disrupts viewing a lot. Therefore, astronomers go to the highest and driest places available for viewing.
Radio Astronomy

Radio astronomy, as we know it, was first started in the 1930s. We credit it to Karl Jansky. He was working on radio communications when he discovered radio waves from an unknown source away from Earth.
Since an abundance of radio waves reach us on the ground, radio astronomy is a growing field. Also, since radio waves are a lot longer than, say gamma waves, the atmosphere does little to them.
In fact, radio waves are the longest form of radiation. Radio astronomy has only begun in the last few decades so we have a lot to learn about these techniques.
We can use radio waves to map out areas in space with much greater detail than using optical astronomy. It’s like this because the atmosphere doesn’t affect a radio telescope. This is very useful because we can not actually see these areas with our eyes.
Radio telescopes have an enormous dish which is their collecting area for the radio waves it captures. It is so large because radio waves are quite rare compared to other kinds of radiation that hits the Earth.
Therefore, we need to maximize our chance at capturing these them. They also operate differently than optical telescopes in that they only see a few wavelengths at a time of radiation.
A tremendous advantage with radio astronomy is that poor conditions mean nothing. For example, if you want to go see a meteor shower and it is cloudy, then you are out of luck. It is the same situation with any optical telescope on Earth.
If there is too much moisture or cloud cover, then we just have to wait until conditions get better. Radio telescopes do not have this problem as radio waves are not affected.
Interferometry
One of the main issues with radio astronomy is the poor resolution. Those telescopes are not near as good as optical telescopes in showing details of an object. This is where interferometry comes in to play. Interferometry is when two telescopes are used together to view an object.
They are linked together to form an array of telescopes. This makes it possible for them to be very geographically separated.
As each radio telescope takes in data, we then combined the data again using special software that makes a superior image than either took to begin with.
When used together like this an interferometer can make brilliant images which get very close to optical images. An important fact to know is that great distances separate the two radio telescopes that work together.
By this I mean they can be on different continents or even further than that sometimes.
A very interesting thing happened as technology and computers got better in recent years. Instead of just detecting radio waves, we can now also detect smaller wavelengths. This opens up our universe even more.
Infrared Astronomy
Infrared telescopes are used to study longer wavelength radiation, and it is a good example of how telescopes are used to study light that we can not see. This is a very popular field.
Longer wavelengths are easier to detect than much shorter wavelengths like gamma rays. A good example of this type of astronomy is the Spitzer Space Telescope. It has given us some truly great images.
This is an important field because it lets us see the universe without the issues of light pollution. Interstellar dust is everywhere out there.
There is so much of it, it makes some gigantic clouds. All of this dust makes seeing difficult. The dust can completely block out a lot of faint objects.
Therefore, studies of the infrared spectrum are valuable. They let us see without our eyes. In the electromagnetic spectrum, infrared lies between visible light and microwave radiation. Its wavelengths are a little shorter than microwaves.
Any object that produces heat gives infrared radiation off. It can be a lot of heat like the sun or just a small amount like the snow. Both still puts off some heat and therefore radiate infrared radiation.
Ultraviolet Astronomy
In the shorter wavelengths, we have ultraviolet astronomy. This is short wavelength astronomy. In this niche area of astronomy, it is ultraviolet radiation that is studied. The wavelengths of this radiation are around 90 to 350 nanometers.
As with all the other areas of astronomy, you can tell a lot about the object when studying its radiation. Examples of what we can infer are densities, composition, and temperatures.
We divided the ultraviolet spectrum up into the near, far, and extreme ultraviolet regions.
One of the cool things about ultraviolet radiation is that the objects with the highest temperatures in the universe give off a lot of ultraviolet radiation. This makes it an excellent source to study.
To study this type of radiation, we usually have to analyze data taken by space telescopes. I should point out that we use space telescopes to gather ultraviolet radiation because the Earth’s atmosphere blocks most of the ultraviolet waves.
Basically, to get to them we must be in space.
High Energy Astronomy
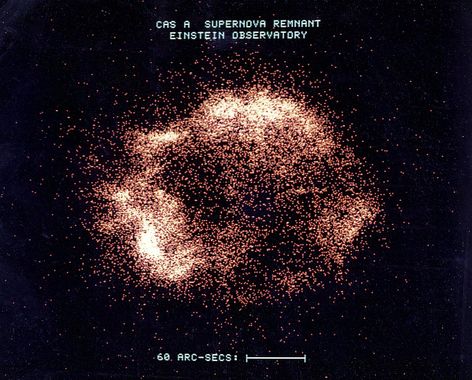
This is the part of the spectrum that is least known to us. High energy astronomy deals with gamma rays and x-rays. They are hard to detect and to capture. Gamma rays, in particular, have the most energy of any other type of radiation.
One of the really cool things about them is that they penetrate everything. This makes gamma rays hard to deal with.
X-Rays
An interesting fact is that the gamma rays and x-rays cover a large part of the electromagnetic spectrum. In contrast, visible light only covers a small fraction of the spectrum.
Just about every day we are discovering more sources for x-rays and gamma rays. The list is skyrocketing. However, there are many more sources for x-rays than gamma rays. In our galaxy, the Sun is the closest emitting source.
Throughout the galaxy there are sources that send forth much more radiation than our Sun. We call these the x-ray binaries which are systems of stars in which one is probably a neutron star.
These objects produce fantastic amounts of high energy radiation. Another super source of x-rays is supernovae which can send the detectors through the roof.
Gamma Rays
Right now there are only about 3000 known sources for gamma rays. That number should explain how rare they really are. As a result, it is more difficult to do a study using spectroscopy because other wavelengths are much more common.
We can sometimes wait a long time before we can detect one. Even more rarely we can observe gamma rays in lightning bolts or certain kinds of nuclear explosions. The typical gamma ray is highly energetic. This means that it contains an abundance of energy.
Its energy is so great that we use it in the medical industry to kill certain cancers. I can’t think of a better way to harness nature for a good cause!
The Astronomer’s Life
Astronomers rarely peer into an eyepiece like they used to. There are a couple reasons for this. One reason is that technology has advanced, and it allows us to do so much more with the data gathered by a telescope.
The other reason is that the atmosphere is degrading which makes seeing objects a lot harder. This is mostly due to all the satellites launched in the last few years. A lot less star light gets to us now.
The light that we can see is often distorted because of our atmosphere.
Most Astronomers spend their time in front of a computer. They are using software to analyze data. They want to use the good telescopes but these have limited time. So they might get a few days a year and spend the rest of the time analyzing their data.
Conclusion
I hope this has given you some inspiration for studying Astronomy. It is a wonderful science and there is much yet to learn. You even have several fields in which to specialize in. Your first step is to get a telescope and start exploring and see where it takes you.
New telescopes are constantly being built. They are larger and more advanced. Hopefully, new discoveries will keep rolling in. I am really excited about the James Webb Space Telescope. It should launch relatively soon.
Important Stuff In The Galaxy
At this point we have a good idea what makes up our solar system. People have been constantly mapping it out since telescopes were invented. So this is a process that has been going on for hundreds of years.
It is called the solar system because that is the area that our Sun is dominant over.
Space objects include:
- comets
- asteroids
- planets
- moons
- stars
- galaxies
- nebulae
- dust clouds
- black holes
All of these are very different and have different origin stories.
One way we know their differences is because we compare everything once we measure everything. It is very insightful.
There is obviously a lot more to the solar system than I can mention here. Certainly each topic is a book itself. I will say that most people get really curious about planets at this point.
Are there planets that we have missed out there? Are there any planets that are exactly like Earth? That alone is an acceptable topic to explore.
Space Debris
If you study different objects, you quickly see that debris makes up a large part of our galaxy. They include asteroids and comets in this group. When celestial size objects collide, stuff flies everywhere.
This is where asteroids, comets, and large clumps of dust come from. How they all interact with each other is just fascinating!
Some asteroids are huge and every so often travel pretty close to Earth. This always makes for big news so watch for these events in your favorite publication.
Comets are fun too. When these get close I highly recommend grabbing your telescope or a good pair of binoculars. These are amazing to see and even better done with friends. These parties are great.
You may not think about it, but dust clouds play big roles in the galaxy. It is often the formational material for stars and planets. It’s the glue, so to speak.
Vast clouds can also blot out the sky in certain areas. A gigantic cloud can just absorb all the light and make portions of space look like nothing is there.
Star Properties
Stars are wonderful objects. Our own Sun is the closest star to Earth. Studies of it and others are very involved. They each have their own:
- size
- orbital velocity
- rotation
- temperature
- brightness
Different temperatures usually imply a relative age. This is because stars usually have an evolution to follow. Different stages usually involve certain temperature ranges.
The size of stars is also very interesting. It is interesting because often that can tell us how it formed. Once we know how a star formed we can infer many other facts about it. Is it in a binary system?
Is it still growing through an accretion disk? Any question we can successfully answer leads to even more knowledge and questions to explore. It can be a deep rabbit hole indeed, but it is so very fun once you get started.
Star Formation
So now you may wonder how stars formed. That is an excellent question and is another area of study in Astronomy.
To put it as short as possible, stars form in the middle of huge gas and dust clouds when the cloud’s own gravity becomes very strong.
Much of the gas and dust is condensed and they generate so much heat that nuclear fusion happens. There is an immense amount of pressure in the middle of new construct and we can now call this a protostar. I have skipped a lot, but that should give you an idea.
Shockwaves are often the start of star formation. They often form right in the middle of the now compressed cloud. They then travel through the cloud in many directions at once.
This action will further compress and heat the material inside. This is so catastrophic to the cloud that it will separate into distinct parts. These distinct parts will now usually become stars themselves.
Star Explosions
We have just mentioned how stars are formed. What happens when they die? This is another superb topic of study that astronomers have to choose from. When stars explode, we can see nova and the awesome supernovae.
These explosions often involve a binary system. The reason is that one star takes material from the other star. These have been called vampire stars for this reason.
While this vampire star is stealing energy, it gets super hot and dense. Anyway, after a while, one star gets too much material to handle. The pressure has also increased.
The star can’t take anymore. Its core has been squeezed by gravity until it is super tight and dense. Think of our planet squeezed so small it’s like the size of a golf ball.
I’m sure that analogy isn’t exactly right but just pretend with me. So, our golf ball can’t stay like that forever. The force compressing it will eventually give way.
What happens next? Well, an explosion happens next. It is an explosion so violent and large it makes anything that happens on Earth look like a balloon popping in comparison. That is what we know as a supernova!
Neutron Stars
Some supernovae turn into neutron stars. This is a misleading term. It’s not really a star anymore at this point. It is basically a ball of neutrons that defy the laws of physics as we know it.
So, after the explosion has finished, which can take a very long time, all the star’s material will get blasted away. The only thing left will be the core of the previous star.
We call this a supernovae remnant. It can take up the space of a small county here on Earth but have the mass of our Sun for example. This density is beyond comprehension.
Neutron stars can also spin. Some spin hundreds of times a second. Think about what has to be going on in the inside for something with the mass of our Sun to spin hundreds of times per second.
Truly incredible in my opinion. Neutron stars that exhibit this behavior are called pulsars. I’m sure you might have heard of them.
Magnetars
Astronomers are still trying to discover more about magnetars. There have been very few discovered. Astronomers have also had a hard time catching them in action.
Their bursts are very sporadic. It has a magnetic field around a thousand times greater than a typical neutron star.
They are about the same size and mass as a neutron star but the difference is the magnetic field. Many stars have only a slight magnetic field.
So we do not know why some of these stars, namely magnetars, have magnetic fields thousand and millions of times stronger. It is surely an interesting area of research.
Magnetars are also a source of x-ray and gamma-ray bursts. These bursts are formed by starquakes on the surface which disturb the magnetic field. There are some theories about why the magnetic field is so strong but I will say that I don’t understand them yet.
They involve super dense fluids after a neutron star forms. I need to read up on them more but there is also very little literature to read, which is the problem.
Black Holes
We do not know a lot about black holes. For the longest, they were just theoretical. Now we have more evidence about them, but not much. They are really just a super dense neutron star with gravity so strong that it sucks everything into them, even light.
Objects would never disappear into a black hole. The gravity is too strong, it would disintegrate the object into atoms before it even got close.
There are many what-if scenarios that scientists have conjectured. Not much has really turned out to be true yet as getting any information from a black hole is incredibly difficult.
The only exception to that is Astronomers think a black hole can’t be larger than 3 solar masses, relative to our own Sun.
Orbital Motion
The motion of planets and other space objects can seem strange. Hardly anything moves in a straight path. Planets and moons sometimes disappear. Even when you track something, its path is somewhere between circular and totally random. Everything is just counter-intuitive.
The reality is really not that bad. Once you know a bit about orbital motion and gravity, it will all make sense. I promise! So let’s get going and restore some order to our night skies.
The most important concept that we need to talk about is gravity. It is the reason for being. It is the key to it all.
Let me say right here that I am discussing the original interpretation of gravity and not Einstein’s curvature of spacetime.
Planetary Motion
Let us start with Tycho Brahe. He was a Danish astronomer who observed the motion of celestial bodies for many years. He recorded all of his data. This data was essential to Johannes Kepler and his eventual laws of planetary motion. His laws of planetary motion catapulted modern science forward.
A planet such as our Earth, Mars, or Venus moves in an orbit. An orbit is the path it takes. Astronomers first thought the orbits of planets were big circles, but they soon discovered it was something else entirely. Planets just disappeared. They disappeared because scientists at the time were looking in a circular path. They did not know about elliptical motion.
Ellipticals
This was the crux of the times. Kepler, however, soon found out that planets moved in an elliptical shape. He did not know why.
We can describe an elliptical as an irregular circle. There is not any definite shape to an elliptical because its lengths can vary. I can call any of the lengths an elliptical. The center of the elliptical to the longest side is called the semi-major axis. It is half the length of the diameter.
The eccentricity of the ellipse is also important. It is a measure of how circular or non-circular an ellipse is. We calculate it using a ratio of the distance between the foci to the length of the major axis. You can think of it as a triangle. You have the center of the ellipse, the foci in one direction, and then the major axis at the other end.
An eccentricity of 0 means the shape is perfectly circular. As eccentricity approaches 1, it is very elliptical. If eccentricity is over one the object is probably not orbiting the center of the ellipse at all.
Newton’s Laws
This leads us to Newton’s laws. He was famous for his three laws, and they had a tremendous impact on the scientific world.
First Law = Every object continues to be in the same state of moving or non-moving and at a constant speed in a straight line unless another force changes this.
Second Law = The change of motion of an object is proportional to and in the force's direction acting upon it.
Third Law = For every action, there is an equal and opposite reaction.
These laws are pretty straightforward. However, let me explain to anyone that might not be familiar with them.
The first law says that objects will stay at rest or continue in the same line of motion unless some external force acts upon it. An example of a force that would change it could be friction or another object striking it. In either of these two cases, the original object would experience some change of motion or direction.
The second law is a little more vague. In a vacuum, this is what happens. However, hardly anything is in a vacuum, though space can sometimes be close. If you neglect friction, a pool ball can change its course to match perfectly the direction that another ball strikes it. The original pool ball will have the same direction and velocity as the one that hit it. Keep in mind, this is a vacuum instance we are talking about.
I think the third law of Newton was his most profound. If you think about it, it is true whether or not in a vacuum. The reason it is so important is that it implies their forces do not act alone. If there is an equal and opposite reaction, that means there are multiple forces at play.
Momentum
This brings us to the next concept that is important in understanding orbital motion, which is momentum. Specifically, I am talking about angular momentum. Let us use planets as example pieces. Angular momentum is its rotation as it revolves around the Sun. It is calculated by multiplying together its mass, velocity, and the square of its radius.
Another factor that Newton’s third law is talking about, is that momentum must be conserved. This is the equation for angular momentum.
angularmomentum∝mass∗rotation∗radius2\]
You can see that mass is involved and that will become more important later on. This is because we must conserve linear and angular momentum at all times. So, with any change to the object, the properties just shift so that the momentum is conserved.
Gravity’s Effect
Now we come to the culmination of our previous concepts. We talked about a force would have to affect any object in order for it to change its velocity. In space, this force is usually gravity. Gravity affects everything. It’s affect depends on the mass and distance of the objects in question.
Gravity is the reason the Earth and the other planets orbit around the Sun. The Sun is so massive that it influences everything in the solar system. All the celestial objects that include planets, moons, asteroids, comets, and gas clouds are all controlled by our Sun. This is what almost all stars do throughout the universe.
The equation that lets us calculate the force of gravity on certain objects is:
Fgravity=GM1∗M2R2\]
This tells us the gravitational force between these two objects. R is the distance between these objects. G is the famous gravitational constant.
Gravitational attraction affects all forms of mass. Since nearly everything has mass, it is ubiquitous. The more mass an object has, the more gravitational force it applies.
Gravity gets weaker with distance, no matter the mass of the original object. However, if the distance is far enough away, the effect is minimal and not really noticeable. That is just the nature of mass and gravity.
Asteroids And Comets
There is an abundance of asteroids and comets in our galaxy. Most were probably formed as our galaxy was birthed. Now, they don’t really have a purpose. They are just chunks of leftover material that got caught in the Sun’s orbit.
Their orbits are very eccentric. Most travel around the asteroid belt. The asteroid belt is a band of rocks(not a rock band) that orbits the Sun. Many have collisions and take trips through the galaxy, even though still fully under the influence of the Sun.
The asteroid belt is between Mars and Jupiter. Many astronomers study this area. They hope to find new and interesting objects, possibly another planet that is hiding there.
Comets usually have much larger orbits. Their paths are very eccentric through the solar system. There are several famous ones that come our way every once in a while. Take the chance to see them when you can. Many you will only see once in a lifetime.
Why Does The Earth Support Life
The Earth is an averaged sized planet in our Solar System. Since it is one of the inner planets, it comprises heavier elements. The Earth’s orbit is nearly circular. It is also the right temperature to keep water on its surface. This temperature means it is not too hot or cold.
Interior
We not know a lot about the interior of our planet, but we can make some good guesses. Our planet comprises metal and rock, mostly. The majority is solid, but there is a portion on the inside that is a liquid. This liquid is molten rock near the center.
The rock layer closest to us at the surface is called the crust. Crust comprises basalt rocks and covers over half of the Earth’s surface. A majority of this is under the oceans and is very hard for anyone to study in those areas.
The layer beneath the crust is called the mantle. It is much thicker than the crust and is denser as well. It is mostly solid but does contain pockets that are molten.
Finally, we have the core at the center of the planet. It is even more dense and hot there. It is mostly solid and is the size of smaller planets. The core is made up of metals and dense rock.
Since the Earth is divided into sections, most think the core was entirely molten. If it was molten, this would allow the heavier elements to sink toward the center core.
Magnetic Field
Our planet has a decently strong magnetic field. It affects a lot throughout the interior. Just like in stars, it formed the magnetic field from hot and dense metal moving in the center. Our magnetic field extends away from the Earth’s surface into space. It traps a lot of dangerous radiation there that would otherwise hit the surface.
The region where the magnetic field keeps out these particles is the magnetosphere. It protects us and is vital to our survival on Earth. These dangerous particles usually come from the Sun with the solar wind.
The Crust
There is a lot going on in our crust. Volcanic eruptions created a lot. Erosion by wind and water constantly reforms it. The Earth is the most geologically active planet that we know of. The crust is made of basalt, with the remaining portion being granite.
Tectonics
Plate tectonics describe the movement of rock in the mantle. These large sections of rock creep along. They have been doing so since the formation of this planet. This movement forms mountains and other large planetary features.
While plate tectonics is often the domain of Geology, it plays a role in Astronomy as well. This is because formation of other planets in the Solar System and beyond must go through many of these same processes. By learning about our own planet, we can surmise details about other planetary spheres.
Tectonics cool the planet. If there is any purpose to it, then it is this. Heat originates around the core and must go somewhere. The shifting of mantle sections brings this heat to the surface.
We divided the crust up into several plates. These plates are below the oceans and landmasses on the surface. They all fit together, but are constantly moving. In fact, the continental structure of our planet is continually evolving. Some plates are moving away from the ones closest to them, while other plates are moving toward their respective partner plates.
The plates move through the convection system. Convection is the transfer of heat which causes cooler material to sink and warmer material to rise.
When the plates run into each other, mountains form and other large changes occur. We can describe these interactions of plates in a couple ways. They are rifts and rises.
A rift is where plates are getting farther apart and causing continents to move away from each other. A rise is the opposite effect. This is where plates are getting closer and their respective continents are being pushed together.
Earth’s Atmosphere
Most of our atmosphere, by mass, is concentrated near the surface. We live at the bottom of the atmosphere. The troposphere is here and is another example of convection. Warm air rises and while cooler air is forced downwards.
In the troposphere, the warmest air is near the bottom, while the coolest air is at the top. Above the troposphere is the stratosphere, which is freezing and moisture free.
At the edge of the stratosphere layer is the famous ozone layer. This is another form of oxygen and it has a different chemical formula. Since it is denser oxygen, it is very effective at stopping radiation from outer space, harming us here on Earth.
The atmosphere consists mostly of nitrogen and oxygen. There are a few other trace amounts of gases. These gases form a fine balance for our living conditions. If there were less or more of them, life would not be possible in the way we know it currently.
We do not know how our atmosphere originally formed. There are guesses involving impacts from outer space, volcanic activity, and formation along with the surface. There is evidence of them all, so deciding on one is not feasible right now. So, most think it is a combination of these events.
Earth’s Weather
Every celestial object with an atmosphere has weather. Weather is the circulation of our atmosphere and its effects on the surface. The Sun plays a significant role in our weather patterns. Its radiation heats the surface, which gives the atmosphere energy.
Rotation of the Earth plays a role as well. As our planet spins, different areas get direct sunlight. This will create weather patterns unique to those particular areas. Weather has the same effects year after year in regions. This gives rise to a climate that does not change much when looked at for long periods of time.
Climates change, though. Change often takes thousands of years for permanent effects to take place. However, change has happened continually on Earth for its multi billion year history.
Therefore, we should not be surprised that climates change and different regions shift its weather patterns. For example, oceans became deserts and vice versa. This has happened many times in our history and will happen many times more.
Life On Earth
Since the Earth is the only place we have identified life, scientists have been trying to figure out why this is. Life has been here since our planet’s very beginning stages. We do not know how long life has been here, but it’s estimated to be around 4 billion years.
Why did life form here but in no other place in our Solar System? There is not an answer for that yet. Many planets have similar formations. We can assume close to the same conditions in countless other planets in other Solar Systems too.
Our atmosphere and surface were much different 3-4 billion years ago. There was very little to no oxygen early in our history. Despite this, life has consistently grown and and become more sophisticated.
Why Is The Sky Blue
The sky is blue because of the scattering throughout our atmosphere. Specifically, light is scattered through air and dust molecules. Scattering is where radiation is absorbed and redirected. When radiation is redirected, blue light is scattered easier.
Blue light is scattered easier because its wavelength is on the smaller side. Since it is smaller, it gets distributed through the air more. This makes our sky blue.
This effect is also why the Sun appears yellow or orange, depending on time of day. In the early morning or late evening the Sun will appear orange. This is because radiation of the Sun has a lot more atmosphere to travel through to reach our eyes than midday.
Since there is more atmosphere to travel through, the blue wavelengths are almost totally gone or scattered through the atmosphere by the time that radiation reaches our eyes. Being gone, all that is left are the other wavelengths. This makes the Sun appear orange to us.
The Moon
It is pretty close to us compared to anything else in our solar system. It's average distance is around 384,000 km but that will vary slightly depending on where it is in its orbit. Radio telescopes and powerful lasers are often used to measure the distance from us to our only natural satellite. No matter how close it may seem though, it is really farther away than most people realize. It is just the closest object to us by far and that makes it look closer. One interesting fact is that the Moon is getting further away from us by 1.5 inches per year on average. Someday that cosmic behavior will affect us here.
How Long Does It Take To Get There
It takes a decent amount of time to get there and that is on a fast flying rocket. Remember it is 384,000 km from the Earth at some times in the year. Originally it was a 3 day trip but subsequent trips have decreased our rocket time to a matter of hours. The New Horizons probe only took 8 hours. Newer ships will be even quicker. Everyone is hoping that the time it takes to get there will be quick enough for regular trips. We will have to see on that!
Characteristics Of The Moon
It's radius is about 1700 km which is a lot smaller than here on Earth and it means less gravitational influence as well. That means the Moon is only around 1/4 the size of the Earth. The average density is 3300 km/m^3 and is also quite a bit less than Earth's density. Why is the Moon so much less dense? Being less dense suggests that there are a lot fewer heavy metals under its surface. Metals such as Iron and Nickel are extremely dense. Gravity is also a lot less on the Moon because it is not near as massive as the Earth. For example, Earth's gravitational field is 6 times stronger than the Moon's field.
There is no atmosphere on there either which is due to its low gravity. Wildly changing temperatures, because of the Sun's intense electromagnetic radiation, are a fact of life since it does not have any real atmosphere. Temperatures range from 100k to 400k and that is below freezing to hotter than boiling.
Surface Properties Of The Moon
The surface of the Moon has been battered by meteors non stop since its existence. The meteors have caused huge craters and cracks in the surface as some of the impacts have been enormous. The Moon has large cliffs and mountains because of this and its surface is changing still. Ancient volcanoes have also helped form its surface appearance. The volcanoes are no longer active but their effects are still easy to view in the large flat plains. These flat plains look quite eerie with their smooth surfaces. They contrast with the rest of the beaten surface in a magnificent way.
Always The Same Side
We always see the same side and you probably wonder why that is. There is a simple reason for this. It rotates on its axis in the same time as it takes t complete one orbit around the Earth. That reason is why we only see one side and there is the dark side of the moon that you hear about occasionally. While that is cool it also means studying the other side is difficult unless your in space.
Rotation Of The Moon
The Moon rotates just like we do even though it does not look like it from Earth. I mentioned above one section that we always see the same side of the Moon and that is because our orbits are synchronized. An orbit that is synchronized means that it's rotation period is the same as its rotation around Earth. It rotates around the Earth every 27.3 days.
Your next question is probably how could this happen to where the orbits are synchronized so closely together. It is due to two things and they are time and gravity. Time and gravity are probably the two most influential factors in the universe.
Earth's gravity has been pulling at the Moon for a very ling time. At first the orbits were not nearly so close. Over time with the Earth constantly nagging and pulling at the Moon it eventually just conformed pretty closely. Now that I think about it, the Earth sounds just like a woman I know...I'll probably get in trouble for that comment.
The Moon's Atmosphere
Does the Moon have an atmosphere? No, not really, it doesn't have one to speak of at all. The reason why is that there is very little gravity exerted by the Moon. Earth's gravity, for example, holds our atmosphere in place which lets us breathe air. Without it, we would would not survive and its as simple as that.
It does contain a few gases that have not escaped but they are in quite small amounts. What we can detect is mainly Helium and Argon but there are trace amounts of other gases too. Gases that are there come from the solar wind for the most part but there are just not many gases on our Moon.
Composition
Our Moon's composition is basically one big rock, or more accurately, many tiny rocks just stuck together. These tiny rocks have been mashed together by gravity and other impacts for a very long time. Remember that it is not very dense and there is a major lack of heavy metals. It also does not have wind and water erosion like we do on Earth. So with all the meteor impacts it has we just see hole after hole on its surface. Some are very small and some are massive.
There is some debate whether it has a solid iron core or not one at all. The consensus is that if it does then it would be pretty small. We already know the density is a lot less than here on Earth so whatever iron it has is going to be tiny.
Moon Dust
The Moon is covered with dust. It is not only covered but it is very thick too. The amount can vary between 20 meters and 100 meters. That is a lot of dust and it shows no sign of going anywhere. The reason there is so much dust is because of all the meteor impacts. After meteors hit the Moon's surface they just destroy everything remotely close to it. Other rocks nearby are ground into dust and this is where all of the moon dust comes from.
Volcanoes
There are volcanoes on the Moon! They are the reason for many of the craters however they may all be dead volcanoes now. Early in the Moon's history there were probably lots of surface volcanoes but for some reason geological activity stopped at some point. The volcanoes were very active at one point as we can see from the significant plains and smooth surfaces.
The Interior
The inside of our Moon is not very dense and in fact it is just about the same density as regular rock. This means that it most likely does not have very many heavy metals like I mentioned above. The composition is likely very much the same throughout.
No Magnetic Field
There is no heavy metal core and therefore no substantial magnetic field. Magnetic fields probably require an iron-nickel core under high pressure and heat. There is none of that and most likely never has been one. There is a tiny magnetic field but it is so small that it basically has no effects.
Origin of the Moon
I hate to say this but we don't really know where our Moon came from. There are many theories and a few really do make sense. They can't all be right though so what we have left are best guesses.
- Moon and Earth formed separately but close together
- The Earth captured its Moon when it got too close
- Our Moon formed from the Earth itself
- The Moon formed from an impact
Based on the little evidence we have I will have to say I don't know where it came from either. The above points all make a little sense but there is no theory that is just right.
Moon and Earth Formed Separately
This used to be a popular theory until we had a better understanding of planetary formation. This theory says that the Earth and Moon both formed around the same time in the same region. This is plausible because the Moon is in our orbit which is a hard thing to do. It does not make sense when you remember how different the makeup is for each object. The Earth has an iron core and many other metals scattered throughout. The Moon has virtually none and its entire makeup is closer to a big rock.
The Earth Captured its Moon
This was another popular theory at first and made a lot of sense because many planets capture their own moons. However, the one thing that stands out here is that our Moon is much too large for this to happen the usual way. Our Moon is around the largest non planet in our solar system and Earth is far from the largest. The gravity of Earth is just not strong enough to capture something the size of our Moon.
Our Moon Formed From The Earth Itself
There is a huge hole in the Earth's surface and it is the pacific basin. This theory says that a young Earth could have been spinning so fast that part of it spun off into space and then became our Moon. The density is similar between the rock in the pacific basin and our Moon. The main problem here is that there is no reason for the Earth to be spinning that rapidly nor is there any evidence of this ever happening.
The Moon Formed From An Impact
This is the most popular theory currently. Remember theories can change in a heartbeat when we find new evidence so it is important to keep that in mind. This one does make a lot of sense though as we have pointed out the flaws of the other theories above. Did this really happen? I don't know. It could have though and that is about as much as we have to go on. This theory says that a large impact by something similar to another planet did a glancing blow that knocked loose a large portion of the Earth's surface. It does at least make sense since the material would be the same and that would make the orbit problem resolve in a nice way.
How Is Distance Measured
The distance to our Moon was originally measured by using geometry and measuring the length of shadows. This was done pretty accurately because they knew the Earth was round by this point. We use lasers and bounce them off a reflector that is currently on it that was put there by astronauts.
Primary Source Of Erosion
Erosion on the Moon is different than on Earth. Really small meteors that constantly bombard the Moon are what erodes it. Since there is no atmosphere they do not burn up but instead constantly hit the surface. However, this process is much slower than erosion on the Earth. On this planet the wind and water erode objects much faster.
Conclusion
The Moon has been up there in our sight for all of our short human history but it is amazing how much we still do not know. We are finding out more details steadily and hopefully we will advance our knowledge at a quicker rate than before.
This is my short guide to Mercury. In it, I discuss its major attributes and origin.
The Cratered World Of Mercury

Mercury is very much like our Moon. It is a heavily cratered body with no atmosphere. It is the nearest planet to the Sun. It gets very hot since it spends a lot of time close to the Sun in its orbit. Its orbit is quickest when it is closest to the Sun. By that same logic, its orbit is slowest the farther away it gets from the Sun in its orbit. It is easiest to see when it is far away from the Sun.
Its Structure
Its mass is much smaller than our Earth. It is around \( \frac{1}{18} \) the mass of the Earth for reference. This makes it the smallest planet in our solar system. However, it is pretty dense for being so small. Its density is \( 5.4 \frac{g}{cm}^3 \). This tells us that the core of Mercury must be made up of heavy metals. This makes it very interesting for us to study. The most likely composition of its core is some mix of nickel and iron. This probably accounts for much of its mass and density. A small magnetic field has been detected so at least part of the core should be liquid.
Density = \[ \frac{mass}{\frac{4}{3}\pi(R^3)} \]
Rotation
Rotation is how long it takes something to rotate. The rotation of Mercury is 59 days. This makes for a very long day! The effect of this long rotation makes for wide temperature changes. Another unique attribute is that a day on Mercury is around \( \frac{2}{3} \) of a year on this same planet.
Surface
The surface of Mercury has been mapped by a couple different spacecraft, Mariner and the Messenger. Its surface looks very much like our Moon. It is deeply cratered all over. It used to be geologically active. We can infer this by the presence of old volcanoes and its partially liquid core. However, there does not appear to be any plate tectonics happening.
Origin
Mercury is composed of a large percentage of metals. It should have a similar percentage of metals and silicates to that of Earth, though. There is no definite consensus of why Mercury is like this. Most people knowledgeable about this topic think large impacts broke large pieces of its surface off or the Sun’s heat evaporated the more sensitive silicates away. It could also be a combination of these two scenarios.
The Rocky Planet Venus

Venus is a geologically active planet and quite interesting. It is interesting because it is one of the closer planets to us on Earth and fairly easy to study. It is a pretty bright planet in our night sky. One of the reasons it is so bright is that it is relatively close to us. So, it shows up nicely! You can check it out either in the early morning or the late evening.
Appearance
We have already said that Venus is pretty bright and therefore, easy to see. In fact, early astronomers noticed it has phases like our Moon. Unfortunately, Venus has a very thick cloud cover. This prevents the surface from being seen.
Rotation
Since we cannot see the surface of Venus, it is harder to calculate rotation. We have to use radar signals. Astronomers bounce these radar signals off Venus and the time until arrival lets us calculate rotation. The rotation period is around 243 days, so quite long. The other interesting fact about Venus is that it rotates backward.
Geology
The surface of Venus is very similar to Earth. There are large flat areas and mountainous areas too. There is no water or ice on Venus and wind speeds are low. It is very volcanic in nature. It appears there are no plate tectonics, which is another interesting feature. This is interesting because there is convection and great pressure below the surface. This usually causes or indicates tectonics.
There are many impact craters. Most craters are 10 km in diameter or more. Most objects smaller than that burned up in the atmosphere. So, this gives an indication of a planet’s age. The most impact craters are, the older a planet is. This is still a rough way to estimate age but it is still useful.
The average surface age is between 300 and 600 million years old. This indicates there is constant volcanic activity and it is very widespread.
Volcanoes
There is continual volcanism on Venus and it has been going on for a very long time. This renews the surface on a regular basis. Volcanoes are an outlet of the mantles' convection and there are thousands of them. When these volcanoes pop, lava goes everywhere. Most of the surface is covered by this lava.
Atmosphere
The atmosphere of Venus is very thick. It makes everything look red. This is because the atmosphere blocks visible blue light particles. It is mostly hot and dry with a small wind. It is about the same across the whole planet. Carbon dioxide accounts for 96% of the atmosphere. Talk about greenhouse gas buildup.
Temperature
Venus is hot, very hot. It is around 700 K. That is extreme heat and is caused by the buildup of greenhouse gasses. This is why we need to get Earth’s environment under control, or this will happen to us and all life will be extinguished. Carbon dioxide cannot escape the planet because of the thick atmosphere. So, it just sits there and contributes further to the heat problem.
Mars, The Red Planet

Mars is a fascinating planet! Seen through a telescope, it is easily recognized. In fact, it is probably the most studied planet other than Earth. It has polar ice caps and other interesting surface features.
Rotation
Mars has a day very similar to ours on Earth. It is 24 hours and 37 min. This is an average because Mars has been observed for well over a hundred years. Mars also has its own seasons. Seasons are a consequence of a planet tilting as it rotates.
Atmosphere
The atmosphere of Mars is mostly carbon dioxide. It’s around 95%, to be precise. However, it is much colder than Venus is. It is actually cold there a lot. There is very little oxygen on Mars and the air is very thin.
Surface
The surface of Mars has a lot of impact craters. It has volcanoes, canyons, and ice caps. It also has many channels that most think were cut by surface water. This is very exciting and is why we hope some type of life might be found there.
There is just about constant wind there. It ranges from a light breeze to a raging windstorm. These storms strip the surface of much of its soil. Bare rock is left with little evidence of the red dirt that was there before.
Much of Mars’ surface is volcanic basalts. These are caused by lava flows that stretched out over great distances. In fact, half the planet is heavily cratered. Most of this terrain is in the southern hemisphere. Around its equator, there is a region consisting of mostly very large volcanoes.
The polar ice caps look remarkable. However, they are not just water that is frozen. They are a combination of \( CO_2 \), which is dry ice and water ice. The northern pole has the most water ice.
Characteristics
Mars has a diameter just over half of Earth’s. It has a lower density though, which means its mass has more silicates than heavy metals. Consequently, it has a small metal core instead of a large one like Earth’s. There is not a planet-wide magnetic field, but there probably was one a long time ago.
Volcanoes
The plains of Mars in the north are very typical of volcanic lowlands. They are lower density and pretty old. This tells us when Mars had a lot of geologic activity going on. The Tharsis area of Mars has super large volcanoes. Smaller volcanoes dot most of the planet, though. The largest one is Olympus Mons and is truly incredible. I don’t do a lot of pictures in my writing but this one deserves a good one and I have included it below.

It is quite possible that this massive volcano is still active today. I, personally, would love to see it active and what it can do.
Climate
Because there is evidence of rivers long in the past, we can infer that the temperature must have been higher at some point. The atmosphere was probably thicker also. It has the same greenhouse gasses that Venus does, so we can assume they all dissipated away from the thinner atmosphere. When the atmosphere thins, the planet loses the ability to retain heat. This is why Mars is a colder planet.
Conclusion
Most of what we know about Mars is from spacecraft that landed there. It is a very cratered planet. A lot of that has to do with the very thin atmosphere, it does not keep anything out. The south hemisphere is a great example of the meteor bombardment.
The Solar System And Beyond
The solar system is very interesting. There are countless objects wandering around out there. We have learned much from even the smallest things like dust in space. The Solar System is a very complicated and busy place. To learn, we measure everything we can and then compare constantly. That is the fun of it to me. I love looking at data and seeing how objects are different. Our resident star, the Sun, is the dominant force in the solar system.
The area that our sun has control over is collectively known as the Solar System. It includes:
planets
dwarf planets
comets
asteroids
moons
meteors
dust
one star
We have found planets that have ring systems. Saturn is the most famous example of this. Most planets have moons. Some moons are like ours but some are very different, like Titan and Enceladus.
Every year there are cool comets and meteor showers to see in the night sky. You do not even need a telescope to see these, as binoculars work great for this.
Comparing Everything
The way that we learn is by comparing. What is the mass of this planet versus the mass of this other one? To do that, we have to observe characteristics and take measurements in whatever way we can in order to build a profile about planets and everything else. Some of these characteristics are brightness, density, mass, composition, distance from host star, orbits, and many other details.
By doing this, we can logically conclude that two objects with roughly the same attributes were made the same way. This is the foundation of our experiments and theory and very important.
How Does Observing The Galaxy Help Us
This might seem like a strange question. I mean, most people would say it is a good idea to observe. But, how does it help us? When I first encountered this question in school, I really could not come up with an answer that satisfied me.
On homework I just put something generic. I am coming back to it now because I feel it is important and wanted to help others who might have had the same issue.
As we observe stars, planets, or comets in our night sky, we measure everything that we can. Things like how bright does it appear or maybe how fast does it move across the sky. We start with basics just to start somewhere.
After we do that for a while, we get an idea for how things work. It may not be a deep idea yet, but it’s there and it takes hold. When we observe other Solar Systems, we get to test those ideas and see if they hold true to these faraway objects.
Size Of Our Solar System Objects
The size of our Solar System objects varies. The largest mass in the solar system is our Sun. It is also by far the largest thing out there besides other stars. The Sun is 99% of our galaxy, if you measure by mass.
The largest planets are Jupiter and Saturn. They are many times the size of our Earth. The smallest planets are Mercury and Venus. Venus is about 80% the size of Earth but Mercury is only around 40% of our radius.
Moons are much smaller than planets. Our moon approaches the size of some of the smaller planets, but others are much smaller. Asteroids are much smaller still.
Types Of Measurements
As mentioned earlier, there are many attributes that we can measure about the objects in the solar system. Some of these measurements can be made directly but others must be indirectly made, or inferred.
Orbital Period = This is the average time it takes for any object to completely orbit any other object.
Mass = The numerical measurement of the amount of matter in an object.
Radius =Distance from the center of an object to the outside edge.
Rotation period = Time for on object to complete a single rotation.
Density = Calculated as mass per unit of volume.
Temperature = Measurement of an objects temperature often in the unit kelvins.
How Do We Get Data On Solar System Objects
The previous section mentioned several properties that we try to figure out about any object. How do we go about this though? Geometry is key to this. Specifically, the use of triangles and their properties. We can learn much using these simple tools. If possible, we even directly observe any moons, asteroids, or planets that we come across.
Distances In Our Solar System
Distances between the Sun, planets, and the Kuiper belt which is at the edge of our solar system are truly vast. The distance between the Earth and the Sun is 1 AU. That stands for astronomical unit. It is an immense distance and is defined as the distance between us and the Sun. There are around 50 AU to the edge of our solar system from the middle.
Our solar system is often talked about as flat. There is a relatively simple reason for this. It is because most everything that orbits around the Sun does so in the same geometric plane.
More About Our Planets
We often divide the planets in our solar system up between the rocky and gaseous planets.
The rocky planets are those closest to the Sun. Earth is one of those rocky planets. The other three are Mercury, Venus, and Mars. These four planets are much smaller than the outer gaseous giants. The rocky planets have some sort of atmosphere.
Atmospheric conditions are all quite different among the four inner planets though. The Earth is the only planet with oxygen in its atmosphere. There is a tangible surface on all of them too. Surfaces are all different as well.
The gaseous giants in the outer solar system are different from the inner planets. They are the furthest away from the Sun and have huge orbits. The gas planets are mostly helium and hydrogen. These gases are compressed until they become liquids at their cores. The atmospheres are gaseous, however.
As far as we know, there are no solid surfaces on the outer planets. Gravity is immense as you get below the outer atmosphere. It is much stronger than here on Earth.
The magnetic fields on the gaseous planets are much stronger than here on Earth as well. They each have several moons in their immediate orbit. Ring systems are also a common factor among the gas giants.
Interstellar Medium
You might think space was totally empty except for all of those large objects floating around out there. It is not so. There are a multitude of rocks, dust, asteroids, and meteoroids in between everything. Dust forms usually when objects grind together and debris ends up scattered everywhere. Larger objects are created through ancient explosions and collisions that have happened throughout time in our universe.
Meteoroids and asteroids are rocky objects flying around out there. Anything larger than 100 meters in diameter is an asteroid. Smaller objects are meteoroids. Comets are much larger objects. Their compositions are usually different, as they contain a lot of ice.
How Is The Interstellar Medium Important
By doing detailed studies of the material between the planets, we learn more about how the Solar System formed. This is the material that formed everything else in galaxies. Astronomers assume it is the stuff from early in the universe's history. They are most likely right. Since it is from early on, it gives us clues about the early universe and its conditions. We can usually infer many properties then and know a little more about what happened after everything first formed.
The Kuiper Belt
At the edge of the solar system is a huge asteroid field called the Kuiper belt. We named it from Gerard Kuiper who was a famous astronomer. Located past Neptune, it is at the coldest part of our solar system. It is in the shape of a disc. The belt is filled with matter of all sizes. Sizes of the matter range from dust particles to sub-planets like Pluto. It is likely that the Kuiper belt is the source of most of the comets that we see. They come our way because the comets get thrown out of orbit. While a few comets get vaporized by our Sun, many more make it to travel the solar system.
The One Orbital Plane
Almost every object in our solar system orbits or travels in the same plane. I mentioned this earlier but did not explain why. I am getting to that now. With so many celestial bodies in space, you might be tempted to think their orbits and paths would be all over the place. It would be easy to think this because there is just so much out there!
That is not the case though. Almost everything is on the same plane. Obviously there must be a common cause to this phenomenon. There sure is.
There is one object in our solar system that has the influence to accomplish the feat of aligning everything into a single plane. It is our massive Sun. The plane that everything travels about is the same as the Sun’s equator. The overwhelming and crushing gravity of our Sun handles this. There are a few minor exceptions where objects were hit and knocked a bit off path but there are very few of these.
Formation of the Solar System
What probably happened, in a simple sense, is that a large disc formed that had a lot of matter in it. This disc was rotating rapidly and eventually matter stuck together. Over time, some matter broke apart and other matter got larger. The larger pieces grew to be planets and moons. The largest piece of matter was our Sun, that was much different then.
Accretion
These primitive discs are accretion discs. They grow by having matter collide and stick together. Gravity is the mechanism responsible for everything staying together. Small objects eventually become larger matter. The process just continues over and over.
Planetary systems and Black holes have this in common. Each can have accretion discs and grow in the same way. Of course different things can happen in Black holes but I will get to that another day.
Planet Characteristics
I mentioned earlier that the planets closer to the sun were more rocky and those further away were gas giants. There are reasons for this. The main one is temperature. Temperature affects a lot of things in the solar system. In this case, it determines how matter behaves and what elements can form.
So what happens on the planets close to the Sun is that it is too hot for matter to stay together. In ways it is the same principle why we use hot water to clean things rather than cold water. The higher the temperature the easier it is for the water to break chemical bonds of dirt and other matter that may be on your hands when you wash them.
It’s the same principle next to the Sun. Matter exposed to those high temperatures breaks apart much easier. Therefore, only the larger chunks of matter remain. That is why there are only rocky planets close to the Sun and why there are gas giants in the cooler regions of the solar system.
In the outer solar system, that matter did not break apart because it was much cooler out there. Clouds of dust formed and stayed together. Gases stayed together and compacted, forming dense clouds. The early gas giants formed in this way after accreting enough material to form a core. Then gravity started affecting things like it always does.
In short, that is why the inner solar system planets are so much different from the outer planets.
Formation Of The Large Planets
There are different theories about how Jupiter, Saturn, Uranus, and Neptune formed.
One of which implies that the original bodies that would become these planets slowly leeched gas from its nebula. Growing like this would be possible because of a strong gravity field.
The other dominant theory says that these large planets formed directly from the collapsed cloud. Strong gravity caused the cloud of gas to become unstable and break apart.
How Did The Sun Influence The Gas Giants
The gas giants are mostly that, just gas. Hydrogen and helium constitute most matter in them. They got all this gas from the nebula in which they were first born. As long as there is gas, then they will continue to grow.
Our Sun was already forming too. You know every star goes through a series of phases in its development as a star. One of these phases is the [T Tauri] phase. During this time the Sun has incredible stellar winds. Stellar winds are powerful and will blow away the nebula of gases around it.
So when the nebula disperses, planets that grow by leeching gas will stop their growth at this point. This bring up two important points.
If the Sun grows slowly, it will reach its [T Tauri] phase with high winds much slower. This lets the gas giants get a lot larger.
When our Sun grows quickly, it reaches the [T Tauri] phase quickly and disperses the gas. Therefore, planets made of gas will be smaller.
Why Are There Definitions Of Planets
They are so different, that’s why. It just makes sense to divide them up like this. I am not sure who first proposed this idea, but it made sense. Almost every property is vastly different. Because of this, we can compare either inner or outer planets to similar groups that orbit around other stars. Usually we can infer they have similar properties in doing this too.
Movement Of The Planets
There is also an interesting theory that says our gas giants came from far away and not created where they are now orbiting at. This theory basically says that they formed in the Kuiper Belt and moved towards the Sun slowly. The reasoning is that the gases that are now on the Jovian planets could not have gone through the planet formation stages at high temperatures. So some think they formed way out in the Kuiper Belt.
Where The Water Came From
It has been long understood that the water in our solar system came mostly from comets. There are certainly reasons that make this plausible. One of which is the frequency that comets occur in our solar system. They are constantly floating around out there. There are issues with this.
One main issue is that it takes a lot of comets to bring that much water. It is true that comets are frequent visitors in our solar system but that is still a lot of water. Once a comet gets close to a planet wouldn’t the friction of the atmosphere burn up most of the water? Even if they made it to the surface, a fiery explosion creates a lot of heat too which would also burn up the water vapor. So there are a few holes and doubts in this theory too, at least to me.
Why Is There Debris In The Galaxy
When people think of our solar system, they often just think of the planets and larger objects. However, most of our galaxy is made of smaller debris. Debris that forms from collisions and other such processes is very common.
Asteroids
Asteroids are small rocky objects that travel through space and orbit around larger objects. They are smaller than planets. Astronomers sometimes call them planetoids. Their orbits are pretty crazy and eccentric.
Most are pretty small. In fact, most moons that we know of are much larger than asteroids. We refer to asteroids as debris for this reason. Since they are so small on average, their combined mass is not much.
Asteroids are usually discovered by Astronomers searching for larger objects. They are usually searching for planets and things like that. When scanning the sky or watching for transits, asteroids will show up in the most unexpected of places.
Astronomers reportedly first discovered an asteroid a little over 200 years ago. Of course, I am sure we saw them, but observers did not know what they were. I am sure they were mistaken for other things in the sky.
Most asteroids that were discovered are in the famous asteroid belt. Almost all the known asteroids are in this region. It is a belt that orbits the Sun and there are many thousands of asteroids there.
Why are there so many in one region? Most astronomers think they are remnants of an explosion that never formed into a planet. This is because there is far too little mass for them to be a broken up planet. They then escape into the solar system. This happens when they collide with each other and they are responsible for most of the cratering seen around the solar system.
Asteroids are a major source of debris in the galaxy. While not very large, there are a multitude of them. They are also easy to find when you want to look for them.
They are usually denser than comets because of their composition. Asteroids are made of more rocky material.
Comets
Comets are another form of space debris. However, they differ greatly from asteroids. Telescopes from far away can pick them up. Comets are almost always brighter than asteroids. This is because they reflect sunlight. Sunlight is reflected because comets are mostly ice chunks and this makes them highly reflective. This also makes them less dense than asteroids because they contain a lot of water ice.
Comets have highly eccentric orbits. Most of us will only see a particular comet once because of their very long travel period. Orbits can be a hundred years to millions of years.
They have a nucleus, coma, hydrogen envelope, and tail. These attributes can all vary in size. Their characteristics all change, depending on how close to the Sun they are. The nucleus makes up most of their mass. When they get close to the Sun, the envelope and tail form.
Most comets come from the Kuiper Belt and the Oort cloud. These are regions left over from the formation of the galaxy and contain millions, if not billions, of comets. Despite their distance from the Sun and our local galaxy, they are still gravitationally bound by the Sun.
Meteors
Meteors are very common and also known as “shooting stars”. They often appear in our night sky as streaks of light. Friction and the Earth’s atmosphere cause these streaks of light. Friction heats the meteor, and this process emits light.
The smaller meteors are probably pieces of comets that broke apart. As comets get near the Sun, parts break away and these become meteors. Their density is very similar to a comet.
However, the larger meteors that make it to the ground are more similar to asteroids. Their density is much greater. So that tells us something else happened. It could be a stray object from the asteroid belt or a fragment from some collision. It is hard to say for sure.
Origins Of Debris
I mentioned the Kuiper Belt and Asteroid Belt earlier. These regions are where most of our galaxy debris come from. Their objects constantly collide with each other and then shoot off in different directions. This is how everything gets scattered around.
The Asteroid Belt
The Asteroid Belt is the boundary between the inner and outer planets in our galaxy. The asteroids contained within are classified into wet and dry objects. We do not know how this asteroid belt formed exactly. There are a few different theories, of course.
One of the leading theories is that the asteroid belt was leftover from the formation of the Sun. This is certainly plausible. Astronomers think some of our large planets came from this left over material too.
Another theory is that this region just filled up with these planetesimals. This is because it was a region devoid of material and rocky objects slowly got trapped by the Sun as they wandered by.
Both theories are equally plausible. It will take more time and data before a leading theory can emerge.
The Kuiper Belt
The other primary source of debris in the galaxy is the Kuiper belt. This is an extensive region of rocks, asteroids, and comets. It starts around 30 AU from the Sun and it is around 50 AU thick. This means it encircles our solar system, and it is quite large. Like the Asteroid Belt, when collisions occur, objects get sent directly into the solar system.
Also similar to the Asteroid belt, the Kuiper Belt is leftover remnants of the formation of the Solar System. Astronomers think it could have come together to form a planet if Neptune had not interfered with its gravity. Its gravity disrupted the Kuiper Belt so often, so the pieces could never come together.
Astronomers think the Kuiper Belt used to be much larger. Our large gas giants have captured much of the material there. Constant collisions have ejected objects toward the Solar System and the surrounding Oort Cloud. The ejected objects are both asteroids and comets, depending on their makeup.
Many of the objects in the Kuiper Belt are large and have their own moons. We do not know exact numbers, but there are probably thousands of such objects.
Radiation
Radiation is the transmission of energy. It can be between any two points or distance. We often apply the term electromagnetic to radiation, and rightly so. This is because radiation energy is carried by electric and magnetic fields. There are many types of radiation, as denoted by the electromagnetic spectrum.
radiation= waves that radiate from a source, like a star.
Light
Light is radiation. We also call this visible light. It is the light we can see with our own eyes. The kinds of radiation or light that we can not see take special instruments to detect and measure. Some examples are radio, infrared, ultraviolet, x-rays, and gamma rays.
Radio - these waves are the largest. We use them to carry radio and tv signals.
Microwave - these waves have a length between a millimeter and a meter.
Infrared - wavelengths between 740 nanometers to 12 centimeters.
Visible Light - these wavelengths are between 400 nanometers to 740 nanometers.
Ultraviolet - these occupy the region of 10 nanometers to 400 nanometers.
X-rays - these range from .01 nanometers to 10 nanometers.
Gamma rays - These are the smallest waves at less than a picometer.
We learn a lot by studying light. Analyzing light can tell us what elements are present, how fast or slow its source is moving, and the source’s temperature. Since we can not visit stars, scientists have to rely on methods such as these.
Waves of Light
All forms of light can travel in a wave motion. A wave is a disturbance of the medium in which it travels. That is the simplest definition I can think of. The medium does not move. It is the energy that moves through the medium. That is a wave.
All waves have certain characteristics that can be measured. These are the period, frequency, wavelength, and amplitude.
Period= time needed for the wave to repeat itself
Wavelength= distance needed for the wave to repeat itself
Amplitude= height of the wave
Frequency= The number of waves that pass any given point in a certain amount of time
The Parts of Visible Light
All light is a combination of different colors. White light is, in fact, made up of red, orange, yellow, blue, green, and violet. These different hues and their resulting frequencies are how we see light.
visible light = electromagnetic radiation that has wavelengths of around 400-700 nanometers.
Each of those colors has a different frequency with red having the lowest and violet the highest frequency. These colors are what we call the visible spectrum. Our eyes can not see any light or radiation outside of these boundaries.
Maxwell and Electromagnetism
James Maxwell was an astounding scientist and, to me, is equal to Einstein in his importance. His theory on electromagnetism is about electric charges and their effects. The electric charges came from particles in an atom.
When charges are opposite they cause motion between the particles. This motion also creates a magnetic field. So, as you can see, electric charges and magnetism go hand in hand.
Now, when particles do not move, as in particles that attract each other, there is an electric field.
I have mentioned both a magnetic field and an electric field. A field is basically just an effect. An electric field influences something else. The same goes for a magnetic field and also a gravitational field.
Maxwell figured out that electric and magnetic waves move at the speed of light. This gave him the idea that these forms of radiation were related. He was correct. We call all of this electromagnetic radiation. It all stems from the same interactions.
Electromagnetic radiation = radiation comprising waves that move through changing electric and magnetic fields. It travels at the speed of light.
Electric Fields
The forces in an electric field can attract or repel each other. It depends on the charge of each individual component. Whether the particle is positive or negative, it will put out an electrical force around it.
If two particles encounter each other, they can either attract or repel away from each other. If the charges of the two particles are the same, then they will repel each other and if they are different, then they will attract each other.
This happens because the electric field of an object extends out in every direction at once. This means it extends out radially in every direction. The strength of this field also decreases with distance.
Electromagnetic Waves
Maxwell was the first to figure out what these waves were. He developed a set of equations to deal with their effects. He combined the knowledge of electric fields and magnetic fields. You get a magnetic field every time an electric field changes.
They change all the time so you actually have a magnetic field all the time too. So, expanding on our wave model of light, these waves are formed from electric and magnetic fields traveling together.
Interestingly enough, they travel perpendicular to each other. Together these two fields make up what we know as electromagnetism.
These waves move at the speed of light which is roughly 300,000 km/s. Of course, this is in a vacuum and with no friction. That is quick, but it is not instant. Of course you could only tell that at long distances. Just something for you to ponder.
Electromagnetic waves do not require any medium to travel through. They will move through vacuum and medium alike. Since that is the case, we can calculate the different aspects of wave motion with this formula:
c=λ×f
That formula means speed is equal to the product of the wavelength and the frequency. You just manipulate the formula, just like most equations, to solve for the value that you are looking for.
frequency = number of waves that travel through a distinct point in a certain amount of time.
wavelength = the distance from the tops of separate waves.
Properties of Light
Heinrich Hertz did the first major work in this area. Hertz’s work built on Maxwell’s, but it was innovative. His experiments showed electromagnetic waves could be detected, and they led to huge discoveries later on.
Light takes the characteristics of both photons and waves. These exhibit both particle and wave characteristics. Scientists conducted many experiments that showed this.
photon = this is a packet of electromagnetic energy.
Photons carry energy. The energy of a photon depends on its frequency. Energy levels of a gamma-ray photon are much more energetic than a photon of visible light.
While photons carry energy, that energy lessens with the distance it travels. Your car headlights are much brighter right in front of your car than a hundred feet away. The light disperses in many directions at once. So, that is why it gets weaker the further away it is from your headlight.
There is an equation that governs this type of phenomenon. It is called the inverse square law.
inverse square law = the amount of light energy moving through an area lessens in proportion to the square of the distance away from the source.
If we go stand in front of the headlight of the car, we will see a certain amount of light. Then if we move three times further away, we will see nine times less light. That is how light propagates and the inverse square law works.
Electromagnetic Spectrum
As you now know, there are several kinds of electromagnetic radiation. Some of it is visible but most of it is not. They have their own characteristics too, meaning we can tell them apart with certain tests.
These differences are wavelength and frequency. So we have to measure these things with instruments and tests. Radio frequencies are on the low end of the spectrum and gamma rays are on the top end.
Almost everything in space emits some kind of radiation. This includes planets, stars, nebulae, and galaxies.
Gamma rays have the shortest wavelengths and so are the most energetic. They are at one end of the electromagnetic spectrum. They are only created in the hottest of stars and phenomena. It takes severe conditions in space to generate them. However, Earth’s atmosphere absorbs them, which is lucky for us because they are very harmful.
The next longest wavelength is an x-ray. This type of radiation still carries a lot of energy. When you get an x-ray at the hospital, you usually have to wear a shield. This is because it can damage some parts of your body more easily than others. You only want the x-rays to hit what it has to so doctors can get the information they need.
Next up are ultraviolet rays. This is also known as black light. It is close to visible light, so that is why it is used in portable devices. Humans can’t see ultraviolet rays. People can see reflections if the objects contain phosphorous. That is what makes objects glow under ultraviolet radiation.
Visible light is the light that we see. It is also the most common type of radiation to get through our atmosphere.
Infrared radiation has slightly longer wavelengths than visible light. They get close to overlapping, just like some others.
Microwaves are the next longest type of radiation. We use them in our ovens. We can also use them in communications. Water absorbs microwaves easily but other substances do not. Therefore, your coffee cup does not burn you when you take it out of your microwave oven, even though your coffee is now hot.
Radio waves are at the other end. They have the longest wavelengths and are the least energetic. There are several categories of radio waves, with different wavelengths. These radio waves can travel great distances. There is even a branch of astronomy dedicated to radio waves.
Opacity of Objects
The opacity of an object is how little light or radiation it lets through. This concept directly affects what we can perceive on this planet. How this affects us is that even in the visible spectrum there will be more opacity and lower amounts of radiation that is let through. Then in other parts the opposite will be true and it will be less opaque.
Thermal Radiation
Thermal radiation is energy given off by heat. Every object gives off a little heat. This happens because electromagnetic radiation is given off at the particle level. This is also directly related to the object’s temperature.
The hotter the temperature, the more electromagnetic radiation is given off. Every object has a heat signature then. This is how you can detect what kind of object you are seeing when you look at its heat analysis.
The type of radiation that is given off, whether ultraviolet or infrared, is determined by the object’s temperature. The temperature can vary wildly from objects in space.
What is temperature then?
Temperature is the particle energy in an object, as they move around. Atoms in every object move constantly, even solids. We just can’t see them. This is because they are too small. It is this motion and movement, at the atomic level, that creates energy and heat.
This temperature and color is how we can identify objects in space. For example, if a star is glowing at a certain color, we know it is roughly a certain temperature. Objects that glow red are less hot than those that glow white or blue. That is just how nature works. I don’t know how or why artists came up with the exact opposite; it makes little sense.
Blackbody Analysis
No physical object emits all of its radiation at one frequency, it is actually scattered around a lot. This is the heat signature I referred to above. Every object will look a little different.
While signatures have a lot in common usually, there are always differences. Just don’t expect them to be radically different because they are not. When graphed this is called a blackbody curve.
Characteristics of Radiation
When graphing blackbody curves of objects you will notice that hotter items shift the curve upwards. Stars are a splendid example of this. Hotter temperatures are higher frequencies too. Therefore, we see objects when they are very hot.
Objects with less temperature or cooler emit radiation in the non-visible spectrum. It is all still radiation though. There is also a simple relationship between the temperature of the object and the wavelength the radiation is emitted at.
wavelength=1temperature
As you might infer from this, the hotter the temperature the greater the amount of energy is involved. This is summarized by the equation:
energy=temperature4
Conclusion
Electromagnetic radiation is the basis for a lot of interesting science. Understanding a little about it will tell us a lot about objects in space where we can not go. Thanks to James Maxwell, we know that charged particles, like electrons, give off waves of energy. This discovery was instrumental in our further progress in science.
The spectrum of radiation comprises many categories. These range from the large radio waves to the tiny gamma rays. Each type has its own characteristics and uses. We also learned that the temperature of the object dictates the radiation it gives off.
Spectroscopy
The study of spectral lines emitted by objects is spectroscopy. Objects emit radiation at different wavelengths and an instrument called a spectroscope analyzes it. Almost all objects will give off and absorb radiation. We know that radiation is just energy. We can not directly see these stars well enough to know much about them, that is why we have to analyze their spectra.
One of the helpful things about spectroscopy is that it is repeatable. This is very important to science. This allows other scientists to duplicate the experiments and get the same results. From there, they can work on the same topic because a hundred people working on the same thing makes faster progress.
Spectral Lines From Radiation
When you analyze spectral lines, you are studying a spectrum of light. It is the division of the radiation’s component wavelengths. This means the light has been divided up. Every object gives off multiple forms of radiation. These will be at different wavelengths. The reason we want to analyze spectra is that it will give us a lot of information about the object that originally emitted the radiation.
intensity
frequency
energy
temperature
magnetic field
When analyzing spectral lines, you will notice they are all very thin. There are differences, but they are small. The lines you get depend on what you are studying. It could be a single atom or a complex object like a star.
The tool used to analyze radiation is the spectroscope. It is one of the core instruments of spectroscopy. Its major component is a prism that splits the light into its wavelengths. There are other smaller parts that help refine the process. You usually look through an eyepiece like on a telescope.
Emission Line Spectra
Emission lines are what we see when we split the radiation into parts. Keep in mind when you do this, only the more prominent parts of the spectrum will show up. This characterizes each object, these distinct emission lines for every object. When we repeat this process, we get the same type of emission lines each time. If we analyze light from another source, then the lines would be totally different.
When looking at an object through a spectroscope you should see black lines throughout the spectrum. These black lines are actually gaps. They represent wavelengths that should be there but are not. Something happened to them so they did not show up. These lines are officially called Fraunhofer lines or absorption lines and are a key ingredient to the study of spectroscopy.
Kirchhoff’s Laws
A German physicist named Gustav Kirchhoff noticed there were similarities between types of spectra. He had a few famous laws.
An object under high heat and pressure gives off a continuous spectrum.
A gas with high heat and low pressure gives off an emission line spectrum.
An object that emits a continuous spectrum and is behind a cool gas under pressure exhibits an absorption spectrum.
Decomposing Starlight
The last couple hundred years the field of spectroscopy has developed quickly and so scientists had several ideas on how to read starlight. One of the most important things that was learned was that the lines on a spectrum corresponded to individual elements. So developing a spectrum for an object meant we could discern its composition.
This is very important because we can’t physically observe stars. So this method allows scientists to determine the makeup of faraway objects. Far away objects are commonly stars. Knowing what makes up a star tells us how it was formed and in what conditions.
When we know under what conditions something it created, we can infer age, size, and velocity. So every piece of information we gather leads to more discoveries.
Atoms and Radiation
Atoms are the basic units from which everything is created. Even at their small size radiation affects them. Atoms absorb radiation which changes the atom. Historically, the electrons in an associated atom were thought to be on precise orbits, kind of like planets.
Scientists now think that it is a very loose orbit around the nucleus of an atom. Some may even be scattered about.
Before modern times we thought everything behaved only like a wave. It made sense most of the time and was assumed to be true for a long time. Eventually, however, our technology improved, as did our instruments and theory.
There was something missing, something not quite right. The answer we now know is that light does not behave purely as a wave. Sometimes it acts like a particle too.
Electrons
Electrons are only found in atoms having a certain transfer. This goes whether they are absorbing or transmitting energy. So the radiation involved in these transactions must correlate to the difference in their separate states. They are known as photons, and are the particles we now know.
A photon is electromagnetic radiation. According to Einstein, the energy of a photon was about the same as the frequency of the radiation. This concept was later worked on in depth by Max Planck and is known as Planck’s constant.
Every element has its own signature on the spectrum.
Hydrogen
The spectrum of hydrogen covers most of the electromagnetic spectrum. When electrons absorb radiation, they then have more energy. Electrons do not keep this energy though. They will lose it. The question is how fast.
If it is quickly lost, then it will emit a single photon with the excess energy but if lost slowly it will emit two photons with the absorbed energy in both. Photons during this process can have differing amounts of energy obviously.
So each photon will be at a different wavelength. Therefore, the spectrum of an object has many lines at different frequencies and wavelengths. The photons that were released after absorbing energy were each given a different amount of radiation or energy.
Carbon And Later Elements
An atom with more protons will have a much more complicated emission spectra. The reason is that there are many more electrons that can get charged and developed into an active state. Each one could get a differing amount of radiation and so give off emissions at a different frequency.
Every different frequency will make the spectra taken from the atom look different. So you can see how different and complicated this can be quickly. Carbon and later atoms of course have larger and larger amounts of protons and electrons.
Molecular Spectra
What happens when different atoms join and make a molecule? Well, things just get interesting. Those different atoms do not stop emitting radiation. They are all doing it at the same time or just a few at once. It depends on what happens with their electrons and what particular atoms are involved.
Since a molecule is just a bunch of different atoms held together by a chemical reaction between their respective electrons, we can expect there to be many more rules about how they interact. The spectra that are emitted from various molecules is therefore a combination of those atoms that make up the molecule.
Analysis Of Spectra
Now that you have sort of an idea of how all of this works, what is exactly is the point you may ask? The very interesting and valuable point is that you use the emission spectra to infer critical information from what you are observing. This can be a star, galaxy, black hole, or planet. Almost everything we know from space is from these studies of radiation that reach us here on Earth.
Composition of Objects
This is another example where the light tells us a lot of information. As light moves through the photosphere of a star, it absorbs the information of things it passes through. These things can be gases or dust, for example.
Temperature Of Objects
Measuring the temperature is one of the important tasks for an astronomer. Knowing the temperature alone gives us a little information but put it together with known atoms and molecules and we get a much better picture of what is going on.
Things emit light and heat because they are hot or emit some kind of heat. Different temperatures tell us approximately what the object is that emitted it. A star that is red tells us the star is on the cooler than a star that is more blue. This makes it handy in identifying objects and their temperatures.
Speed Of Objects
The shifted lines of an object can tell us its velocity. The well known Doppler effect does this. Looking at the source radiation and checking for either red-shifted or blue-shifted lines can tell us a lot. If they are blue-shifted, then the source is moving toward us. Red-shifted lines mean the source is moving away from the observer.
Width Of Spectral Lines
Even the width of these spectral lines can tell us a lot about what is going on. The width indicates the environment of the source. Most often the velocity tells a lot. A higher velocity will usually mean a higher temperature too. Magnetic fields can also broaden spectral lines. The stronger the magnetic field the more broad the line usually is.
Conclusion
In this article, we have discussed spectral lines, and why they are useful. Spectral lines are a field of study, called spectroscopy. This is a very useful field because all light or radiation gives clues about where it came from. These clues include temperature, velocity, and composition of the original source of the light.
Exoplanets
Exoplanets are planets that are beyond our solar system. They have been known for a hundred years or more. They are really hard to study directly. We can still learn a lot from them though.
Introduction
In fact, that is why we study them. Learning about exoplanets will help us learn about the formation of our own Earth and corresponding solar system. Astronomers monitor young planets to see what Earth might have been like in its early stages. In this article I want to talk about what is known about exoplanets and how they are formed. This will obviously lead into solar system formation which is pretty interesting stuff.
History Of Exoplanet Detection
Scientists first thought they had discovered an extrasolar planet in the 1800's They certainly could have been right. We do not know for sure though. Slowly more and more evidence was found that indicated these existed. It was not until more modern times, however, that Astrophysicists were able to verify some of these claims. Now space telescopes like Kepler, Spitzer, and Hubble find exoplanets quite often. Finding them and documenting their properties is key for our understanding to evolve. Its a very important time in learning about our universe.
Formation of Exoplanets
Solar systems and exoplanets form because of stars. A star is a luminous object made of plasma that spins. When a star starts its processes it will be rotating. As it rotates it gathers all the rocky and gaseous material around it. This material will then form into a protoplanetary disk. A disk like this looks sort of like a cd to us. It is flat and it rotates around its star. This is where planets and moons come from, it just takes a while to happen.
Exoplanets that form from this can either small and rocky or giant and gaseous. These extrasolar planets will often move from where they are first formed. They move because of other objects and processes. Collision and gravity from other objects are the main reasons. Collisions can either destroy objects or eject them into space. Observation is key here. Direct observations are the best but indirect will lead to nice results also.
We learn about planets from the huge cosmic gas clouds we can observe. It is from here we learn about these extrasolar planets that inhabit other galaxies. Astronomers have discovered a few thousand exoplanets from the data I can find. The more they are studied, the more we will understand about our own planet and its processes. Currently, we just have very vague data on all of these extrasolar planets. They are so far away in the universe that estimates are the best that can be done. Astronomers are learning more by the day though.
Solar System Formation
The main theory of solar system formation is the nebula theory. Everything in our system formed from gas and dust. It was all one big cloud covering a massive area. The nebular cloud became unstable because of some event. We don't know what kind of event though. Eventually the gas and dust stuck together and formed other objects. The Law of Conservation of Momentum is the reason some of it started rotating. The center started rotating and pulled in a lot of material around it. Everything that converged to the center eventually became a star. The material that was left behind in the disk formed our planets. Rocky material formed our first few planets. The gaseous remains were made into the big gas giants like Jupiter and Saturn. Astronomers now think this is how most solar systems are formed.
In our quest for knowledge, we are constantly refining our views on how solar systems are created. There are many explanations. Some of those seem quite strange today! There are a few ideas that, so far, have stood the test of time. Exoplanets orbits are somewhere between perfectly circular and highly elliptical. This is vague but it is also a starting point. Orbits are usually in the same direction as the Sun's rotation. Random events, such as collisions, do happen and they cause drastic change. There is evidence of many large object collisions in our galaxy between moons, asteroids, and planets. These collisions have shaped and influenced most of our galaxy. I am sure other galaxies have had mostly similar experiences.
Search For Exoplanets
Astronomers are constantly searching for exoplanets. With technology advances, we can finally try to confirm discoveries made many years ago. Now you might wonder how exoplanets are discovered and verified? It is quite true they are so far away we can only barely see a few with modern telescopes. So they are observed by other means. The most popular ways are studying the light from the star it orbits and the radial velocity of that star. Radial velocity, in this context, refers to the change in the velocity of its spinning relative to us here on Earth. It is our perceived changed in an objects velocity far away.
Planetary Transits
When we study the light of stars there are times when the brightness lessens slightly. This happens occasionally and it is often an indication an exoplanet is orbiting this star. When it travels in between our line of sight and the star, the light output decreases. Astronomers are using this easy technique constantly to find new exoplanets.
Radial Velocity
The other technique that is used to find extrasolar planets orbiting other stars is measuring a star's radial velocity. What happens is that periodically, the gravity of an exoplanet will cause a slight wobble in its parent star. When this happens the rotational velocity is measurably less. Just like planet transits, when this happens we watch closely and if it happens continuously and at regular intervals we know its being caused by an extrasolar object orbiting around that star. Astronomy is all about those observations!
Other Methods
We can also just take direct pictures of exoplanets. This is pretty rare though. Astronomers have to get very lucky for this to happen. They are very far away and they are very dim as well.
Another way we can find exoplanets is through gravitational lensing. This is really just the study of light. Light is affected by gravity over long distances. It is bent when a planet passes through its light.
Exoplanet Characteristics
Thousands of exoplanets have now been discovered by our space instruments. Most have been spotted by the Kepler Space Telescope. They have been rocky, icy, and some made mostly of gas. A few have been in the habitable zone of their stars. Some are very close to their star and are tidally locked. A planet is tidally locked when their is a large difference in masses between the two. Distance also plays a factor. When an exoplanet is tidally locked it also shows the same side to the star. An example of this is our moon because it always shows the same side to us here on Earth.
Planets that are much larger than Earth are called Super-Earths. These are planets that exhibit many of the same properties as our planet here. They are assumed to be rocky and fairly dense.
Gaseous planets that are many times the size of Earth and orbit close to their star are called Hot-Jupiters.
Most planets are detected as they transit across our view of a particular star. A few, however, are detected by the radial velocity technique.
One of the problems that Astronomers struggle with is the composition of these exoplanets. Knowing the composition would allow them to be able to guess about their density. Most exoplanets discovered are very hot and gaseous. These planets put off the most light. That makes them the easiest to see. We know that many planets should exist that are like Earth. They are small, like Earth, so they are harder to detect.
Habitable Zones
One of the main reasons we are searching for exoplanets is to find some in habitable zones. Scientists want to find something that has life on it. Some stars will have an area around it that would be the right temperature for life as we know it. That is what everyone is looking for. Another key ingredient to this is the ability to hold water. It has been determined that this is important for life. I wonder how they figured that out.
Finding life will be more difficult. We know what life here is like. That is how the first search started. It was, and still is, looking for signs that organisms like us are out there. Who really knows if we will ever find that. The other problem being tackled is recognizing life that is very unlike us. If it isn't very similar to us we might never know that we saw it. We just don't know how other forms of life could look.
To be successful at all of this we have to stare through space. The problem here is that the light of other stars outshine small planets that might host life. NASA is working on technology to help with this problem. Its goal is to dim incoming light but allow space telescopes to image space better.
Different Planetary Systems
Since there are so many different galaxies, it would be safe to assume that some formed in unusual ways. Ours is one of those. Astronomers have not found a solar system that is like ours exactly. They have not discovered a planet with any sort life on it either. That makes us pretty unique. Systems have been discovered with Jupiter size planets orbiting a Star like ours. Still, its a bit different. Most Jupiter sized planets orbit much closer to the star. They also have more eccentric orbits. Those are some big differences and we don't know why.
Astronomers have also not found another Earth or anything that resembles it. We have life all over the planet including its oceans. There are plate tectonics that have constantly moved. An atmosphere filters out the deadly radiation from the Sun. We even have cosmic protectors in the form of Jupiter and Saturn. Those gas giants grab deadly asteroids that head this way and lock them in their gravitational grasp. As a result, we rarely see anything that hurts us. There has never been anything that collided with this planet and broke it apart, unlike many other objects. So we are very unique.
Conclusion
Exoplanets have been discovered by the thousands now. Some are even relatively close to us. Most that have been found are large, hot, and gaseous. This is because they emit more light and are thusly easier to see. They form in protoplanetary disks after a star starts its processes. These exoplanets will then move around and collide with other stuff. This allows it to gather more material in its physical formation. They are quite varied in size and composition. Rocky, icy, and gaseous are all represented. Different properties such as these have allowed many types of solar systems to develop. Astronomers can't explain why systems can be so different. Everyone is trying to find a solution.
How The Sun Works
I want to start out by saying that our Sun is our only source of heat. Without it, our planet Earth would freeze and us along with it. We would not survive. That is how important this star is to our survival. So what is a star? It is a sphere of extremely hot gas. Strong gravity is what helps the Sun hold its shape and keep all this gas in one place. In the center of the Sun are constant nuclear reactions that keep the engine turning.
Properties Of The Sun
The Sun is quite large by our standards. It's radius was measured to be almost 700,000 kilometers and diameter to be 1,400,000 km. This measurement was made by the SOHO spacecraft a few years ago. It also rotates just like us here. Rotation is different though according to the location on the Sun. It is faster at the equator than towards its poles. This is called differential rotation. Since its rotation is differential, we can infer that the Sun is not solid and is instead gaseous and fluid. Complete rotation takes about a month. The temperature is pretty hot at around an average 5800 kelvin.
The Sun's radiation reaches us here on Earth. Its energy is what gives us warmth and allows us to live. Different amounts reach here depending on the location. The equator gets more than our poles. That is because the equator faces the Sun more. Earth is tilted after all. The poles get very little radiation and is why they are so much colder.
Layout Of The Sun
The layout of our star has been quite organized by those studying it. It is divided into several regions. Those regions are the photosphere, chromosphere, transition zone, corona, convection zone, radiation zone and its central core. These zones all serve different functions. Studying them will explain how the Sun works and allow greater understanding of our universe.
The photosphere is what we see when looking toward the Sun. It is also where most of the light comes from. It takes 8 minutes for the Sun's light to reach us here on Earth and it constantly bombards us.
Brightness Of The Sun
We know that the Sun gives off a lot of radiation. How do we know that? Look at the light during the daytime. Light is the Sun's radiation. Most people do not think about that. We can measure this light as well. There is a solar constant which is the amount of light that hits a given spot of a certain size. At first we thought this was very constant. That is reported anyway. I do not believe it is that constant all the time and that it can vary a lot. When it gets very cold or hotter than normal for a period of years, this is the Sun being variable. This has happened throughout recorded history and is most likely what is happening instead of global warming due to mankind. A lot of the Sun's light does not even reach us. Only a tiny fraction gets through to us. That is important to realize when you consider our hot days during the summer. Only a very tiny fraction comes through space and past our atmosphere.
Standard Solar Model
Since we are not able to directly observe the interior of the Sun we have to make assumptions based off of things we do know. Putting all these assumptions together is called a model. So we have an information model of the Sun that we work from. It comes from a little direct observation and various laws of the universe that are already in place and accepted. Specifically these are the laws of energy, momentum, and mass. Now when physicists put all these things together they are assuming the Sun is a perfect sphere and radiates energy outwardly in an even fashion. The reason this is done is that is because non-uniformity increases the complexity of these problems immensely. Most actually think this is still pretty accurate as well.
Hydrostatic Equilibrium
As we talk about how the Sun works it is important to know about its hydrostatic equilibrium. What this means is that its pressure and gravitational forces are close to equal most of the time. This is actually one of the reasons we assume that its energy output is fairly equal in all directions. Most stars will also stay in equilibrium too. The reason for this is that these forces self-regulate. If one force gets increased by an external factor it can usually balance itself out. This is also one of the reasons why stars and objects like them can be so long-lasting.
Energy Transport Inside The Sun
Since the Sun is so hot near its core, collisions between particles happen continually. These collisions carry energy. Now we know that the temperature drops the further from the core that you get. This is important because electrons will stay with their atoms easier. Electrons carry energy in their excited states. You may see a problem by now. How does energy move to the surface and beyond then? The way it moves is by convection. This is how energy escapes the Sun and is very important as to how the Sun works.
Granulated Cells
When looking at pictures of the Sun's surface you will see many granulated regions. These are huge areas of intense heat. The granulated regions appear to be different colors. Color is mostly dependent on the temperature of individual cells.
Spectral Lines Of The Sun
Spectral lines appear when electrons change states. When doing tests we can use these lines to see the composition of what we are studying. Spectral lines of the Sun can tell us the elements that constitute it. However, these lines also give evidence that the temperature of the Sun decreases as you get away from the photosphere.
Chromosphere
This is a region just above the photosphere. It is much dimmer and a little cooler than the photosphere also. The chromosphere spawns solar storms that sends out spicules into the atmosphere. It is approximately 4000 km deep. The temperature of the chromosphere is also unusual because its area around the photosphere is cooler than at the top of the chromosphere. Usually anything gets cooler the further from its core it gets. So this makes the chromosphere of the Sun quite unusual. Shockwaves from the photosphere move into the chromosphere at a high velocity. This helps in heating the surrounding areas.
The Corona
The corona is a fascinating region of the Sun's atmosphere. Elements arising from this area are in much different forms than anywhere else. Coronal temperatures are much higher on average than the photosphere even. Temperatures of 3-15 million k are common here. That is extraordinary. Intuition says there must be something else causing the heat instead of just the core. It is not clear what this mechanism is though.
For all of its heat, it is not very bright. We normally see it during eclipses. Magnetic forces move energy into the corona. This produces its high temperatures. The corona constantly leaks into space with the solar wind carrying its particles far and in most directions at once.
Solar Wind
The Sun's solar wind is not really a wind in the sense that we know it. It is more akin to a stream of radiation and particles that have been ejected at super high speeds. As far as we know radiation and particles are ejected outwardly in an even fashion. It all comes from the corona itself because the temperatures are so high there.
Magnetism
Magnetism is one of my favorite subjects. It is no less interesting or relative when talking about how the Sun works. Our Sun has a very strong magnetic field. Its mechanisms and insides follow the electromagnetic laws. Astronomers do not know where its magnetism is created though. Many are still studying this. If I was to guess I would say the magnetic forces are created constantly. The reason I say this is because the high temperatures separate molecules and atoms into individual particles. When these particles are then moved by the abundant energy all around them, they create magnetic fields. Since temperatures everywhere in the Sun are so hot this happens a lot. That is my take on it. I could be totally off but we will see.
Sunspots Within The Sun
Sunspots are dark patches that often appear on the Sun's surface. They are quite large, about the size of Earth actually. Sometimes they appear singularly but also in groups. They appear dark because their temperatures are lower than the area around them. When seen up close they have a darker core and a slightly lighter area around the core.
The sunspots are all on a cycle. Most people think its around 11 years but others think there could be even larger cycles. As we get more data on how the Sun works this will be more evident. What this all means is there are quiet periods and there are raucous times as well.
The Magnetic Field
Magnetic fields within sunspots are pretty strong. They are much stronger than here on Earth. Since they are so strong, they redirect convection currents. This is what makes the sunspots darker and cooler than surrounding cells. Magnetic fields always have a polarity as well. This means the direction in which the field runs. Currents and particles seem to follow the same direction as the field lines. Sunspots in the northern hemisphere have opposite field directions as those in the southern hemisphere.
Cycles Of The Sun
This is still in regards to sunspots but it is still key to understanding how the Sun works. Astronomers have been observing the Sun for hundreds of years. During this time there are a few things they have noticed and passed down to later generations. One of these is that solar sunspots are quite variable and prone to change. Sizes, shapes, and durations of sunspots all change constantly. Our Sun does have a long cycle in which sunspots are more common. It is 22 years. We can further break this down into two 11-year periods though. During each 11-year period the polarities reverse.
Solar Flares
Solar flares are born of the chaos of changing magnetic fields. They produce incredible amounts of energy. Energy is visible in multiple frequencies so we can study them with x-ray and ultraviolet detectors. They are basically very powerful explosions that occur on the Sun's surface.
What Powers The Sun
Everyone knows the Sun gives off lots of energy. The thing is, it continually does this and has done so for a long time. It is common sense that there must be some renewable source of energy and this mechanism is very efficient. This mechanism is nuclear fusion. Nuclear fusions is when two nuclei are joined together. A third nuclei is then formed. However, this third nuclei is smaller than the two previous nuclei. When this happens energy is created because that missing mass has to go somewhere. This is Einstein'sE=mc2law. It predicts the energy released when this happens. This is what is constantly happening in the Sun and is fundamental to how the Sun works.
Conclusion
Hopefully by now you understand some of the basics of our parent star. There are so many fascinating details to study I could not even include everything. There are also newer but untested ideas being presented constantly. It will be quite interesting indeed to see what ultimately develops. I hope that I have shown how the Sun works to a sufficient degree here. If you have any questions please comment below and I will answer. Have a great day!
Stars
Within our own solar system there are untold numbers of stars. If you happened to somehow leave our galaxy then the number of stars increases exponentially. So the first thing we want to do is figure out how far away all these stars are. When we figure out distances to the stars their other properties can also be inferred to a decent degree of accuracy.
Stellar Parallax
Stellar parallax is the degree our view changes when an object is observed from two different points whether from telescope or with our eyes. It is also described as the displacement of a star as seen from Earth. This lets us calculate the distance between us and the object. this is done using angles between the points involved and geometry. The easiest object to practice this on would be our Moon. Distances are measured in arcseconds. The distance an object is away from us that has a parallax of 1 arcsecond is called a parsec, which is slightly over 200,000 AU. An AU is the distance from us to the Sun. Parallax decreases as the distance gets greater so we can use a formula to calculate it.
distance=1parallax\]
Motion of the Stars
The stars move. This may not be apparent, especially if you never thought about it before. They move very fast as well by our own standards. They are just so far away it is difficult to notice. They move across our sky a very small amount in a human lifetime. Stars have their own motion as they soar through the sky and they also rotate extremely quick too. Proper motion is how to describe a star's velocity.
Luminosity and Brightness
Every star has its own luminous qualities and apparent brightness as seen from Earth. A star’s apparent brightness depends partly on its distance from Earth. So, the amount of light that reaches us varies inversely as the square of its distance from the source. Therefore, doubling the distance from a star makes it appear 4 times fainter. This means two different stars can appear to have the same brightness. If the brighter star is farther away than a slightly dimmer star then to us they could appear to be the exact same. Remember how we talked about inferring properties of stars once we know a few things about them? This is a good example. If we know a star’s distance through the use of parallax calculations and a particular star is farther away, but appears to have the same brightness, then we know it is inherently brighter and puts off more radiation than the other star.
Star Temperatures
Stars also have their own temperature ranges. Star temperatures can vary greatly. However, we can get an idea of its temperature from the star’s color. This is not very intuitive but red stars are a lot cooler than blue stars. Astronomers measure the temperature of a star by taking the brightness at several different frequencies. They then match these readings to a blackbody curve and see where they align. This will give the approximate temperature of the star.
Spectra of the Stars
Spectroscopy is a very useful tool. I talked about it in a past article and it has many uses. With stars, it is used to collect data on individual stars and then study the differences. The patterns are what tell us the differences and compositions of stars. Spectroscopy is a fascinating subject and can tell us so much just by looking at absorption lines.
Stellar Size
H-R Diagrams
Mass of Stars
Conclusion
How Stars Form
Formation Of Stars
Interstellar Clouds
Star Formation
Star Clusters
Conclusion
How Stars Evolve
Stars spend most of their lives in the main sequence. Eventually, they start to run out of fuel, which causes changes. Different things can happen at this point. It starts changing from the inside, its appearance changes, and it might explode. Whether a star explodes or not, depends on its mass at the time. Lower mass stars just go out. They turn into white dwarfs. Higher mass stars will explode. If a star has 8-10 times the mass of our own Sun, then it will most likely explode in a fiery wave of destruction. Modern Astronomy has learned a lot in recent years and developed theories to explain why these processes happen.
Life in the Main Sequence
You might wonder how Astronomers can see stars evolve. The trick here is that they study stars that are on different stages of their life. These different stages are how we organize the stars that we see and are called star classifications. They can then piece these together to get an idea of how they evolve from a disk to an explosion. These main sequence stars turn hydrogen into helium. This happens in the beginning phases of the main sequence. It is also important to remember that stars are hardly ever isolated by themselves. They form in clusters and can influence the others in its general region in unique ways.
A Star’s Evolution
When the heat in a star around the size of our Sun decreases to a certain point, the helium in the core will not fuse into any other element. This will make the helium core shrink. Astronomers call this the hydrogen burning phase. The surrounding hydrogen is burning violently. As the helium core shrinks, it gives off a lot of energy. This energy is what fuels the hydrogen burning phase. As a result, the star itself becomes much brighter and hotter. It is now putting out a lot of radiation.
When the helium core has shrunk enough, it will fuse into carbon. The new carbon is not compatible with the remaining helium core. This causes a strange reaction. The helium starts to really burn and results in a helium flash.
The End Of A Low Mass Star
At this point, the helium is burning, but carbon is still forming. The carbon core becomes much more dense and hot. While this happens, the start gets even brighter. We see this and evidence of it happening in the last through spectroscopy studies. Remember, a star is usually in equilibrium throughout its life time. The temperature in the core is the driving force for what happens later on. So when this temperature drops, changes will occur soon. This is all dependent on mass as well. This type of star, which has a relatively low mass, won’t be hot enough to fuse carbon. Eventually its outer envelope is burned away and sent into space as a planetary nebula. What remains of the star is what we call a white dwarf. Over time, the white dwarf just cools and cools.
Large Star Evolution
Higher mass stars take a different path. The reason is the higher mass. This larger mass means the central core of a star will be much hotter. This time, the core becomes hot enough to fuse carbon. They will become red supergiants. This higher heat will cause heavy elements to form. It will also happen quicker and quicker. This will be too much for the star to handle and it will end up exploding! When a star explodes it is a significant event and one of the best ways that our telescopes are used. We all want to see what is happening.
Stellar Evolution
The evolution of stars is getting clearer by observing stars of all ages. They can be in isolated binaries or surrounded in a globular cluster. The massive stars leave the main sequence first. They are followed very slowly by stars like our Sun. Stars in binary systems can evolve in quite different ways. Each in the pair can affect the other in strange ways. It is a weird relationship to be sure. If one of the pair evolves faster than the other, it can lose a lot of its gas and mass to its companion. This will cause a slower evolving star to actually gain speed in the sequence.
Fusion In The Core
Fusion is the effect of the high temperatures and all the processes going on within a star’s core. For a star to keep evolving, it will need to fuse heavy elements, such as carbon. When it successfully does this, it will get to its next stage. From there, depending on its mass, it might explode into a fiery shower of debris. This often can leave a planetary nebula in its place. These are not related to planets of our solar system however. Its just an old term that was wrongly used but stuck with us. However, if the core temperature is not high enough, these processes will not happen. Instead, there will be no more fusion and the core will eventually cool. It will still take a long time, but the cooling effect means it will turn into a white dwarf. A white dwarf star is one that is no longer burning at its core. It does have a lot of residual heat, which can be seen as it glows.
Lifetime Of Stars
This is related to previous points that I have made but I wanted to be more specific. Thee lifetime of a star depends on its mass. This mass was decided early on in its original cluster. The lower the mass, the longer the star will survive. If its smaller than our Sun it will last billions of years. However much of that time it will just be a shell that is slowly cooling off. It will have no internal activity. Its fate is to be a cold, dead, rock, sort of like our moon. However, our moon was never star so don't get confused. In the solar system we live in, this should be the fate of our Sun someday.
A high mass star will have a much different fate. The more mass it is the shorter its lifetime will be. If it is a lot larger than our Sun, then it will only be around for a few million years. That is a great difference. The reason why, as stated above, is that its mass and temperatures are so high that it will trigger a violent explosion. All that will be left, could just be a planetary nebula and space debris. This nebular is just a gas shell that is just a faint reminder of what was once there.
Conclusion
In this article today I have talked about the stellar evolution of a star, which is part of my astronomy essentials series. This evolution is very dependent on its original mass. This mass determines whether the star will end up as a cold dead rock or blow up in small pieces across the galaxy. So, their lifetimes can be very different in actual spans. Their life spans can be tracked, depending on where they are in the main sequence. The main sequence is sort of a guide to the universe for stars because we classify all of them there.
Exploding Stars
Stars explode all the time. They do so for a few different reasons. The type of explosions and aftereffects depend on the characteristics of the original star. As you can imagine, there are multiple types of explosions. There are different types of explosions because there are so many different types of stars.
White Dwarfs
A white dwarf is a star that is no longer progressing. In certain ways you could consider it a dead star. For example, it is no longer burning from the inside like it used to. This means that the fusion process has stopped completely.
It is also very dense. Density has increased because it will have shed all of the lighter materials that used to make up this star. You can still see these white dwarfs because they have some residual energy left over. This is a finite resource, it is no longer creating energy. It will eventually go completely dead.
Nova
A nova happens when a star undergoes a transformation that causes it to brighten considerably. It will then take a long time for it to lessen in intensity. These novae all involve white dwarfs and a larger main sequence star.
When a white dwarf gets close enough to its larger companion star, it will start to steal matter from it. The stolen matter kind of hangs above the white dwarf but very close to it. This gets heated up violently until it pops.
Binary Stars
A binary system is often one of the keys to start the nova process in a white dwarf. The reason is that the second star provides a lot of excess material to the white dwarf, which is usually denser and has more gravitational forces in its center. When looking for possible stars to go nova, astronomers often look at known binary systems first.
Larger Stars
The difference between large and smaller stars is all dependent on whether it burns carbon in its core. Smaller stars, such as white dwarfs, will not do this. Really big stars will burn and fuse carbon along with many other heavier elements. When a large star does this, its central core temperature rises to unbelievable heights.
One of the ways it achieves this is using the remnants of previous materials as fuel. When burning first starts the lighter elements get burned up first. This creates fuel to burn heavier and heavier elements in time. After each of these stages, the star’s central core keeps heating up. These processes happen many times.
Each time it happens there is more heat and pressure involved. The iron core gets disintegrated into lighter elements. Neutrinos eventually form and take energy away with them into space.
Supernova
This is when things get real interesting. The core has reached crazy densities. Our collective understanding of physics can’t even grasp why everything happens the way it does at this time. What basically happens though, is that once the core has reached a certain density, things have to reverse. I mean, you can’t condense something so much that is disappears after all.
So now we have this iron core that is super dense. After a while, it can’t take anymore and it starts to expand. Now expand is probably not the right word because of what happens next. This expansion happens super quick, in an instant actually. It creates a devastating shock-wave that travels throughout the whole star.
This shock-wave is so violent that the whole star explodes and is destroyed. There is so much energy released that the star is as bright as all the stars around it put together. This star is completely destroyed and the only bit left behind is a gas shell. That is something indeed.
It has been a long time since anyone saw an explosion in our galaxy. So everything recently has been supernova explosions from far away.
Supernova Remnants
A remnant is what is left behind after a large star goes supernova. They are the remains of a supernova explosion. There are many such example nebulae that we have nice pictures of. They are quite beautiful.
When these stars explode, material is ejected at very high velocities. This matter in interstellar space gets spread everywhere and it becomes fuel for other processes. This is very important because it is one of the ways that heavier elements get spread through the galaxy. They are supersonic, which is the cause of the shock-waves. This means the shock-waves are moving so fast they travel vast distances. These outward waves sweep up almost all material before them. This process can last hundreds to thousands of years and travel many parsecs.
Remnants can be classified into shell types or hybrid types. Shells can be characterized by their ring-like structures surrounding them. There is more hot gas at the edges and that is where the bright rings come from. Hybrid types usually contain a pulsar. The pulsar creates many high energy particles such as x-rays and and radio waves.
Eventually the remnant gas shell cools. Relatively speaking the shell is quite cool and dense. It can then last a very long time. There can also be hybrids that contain a pulsar. These pulsars are some of the main sources of gamma rays. They are also called high energy.
Conclusion
In this paper today we have talked about why the stars explode. This is astronomy essentials at its finest as we shall see. Smaller stars will result in a nova. This is because they do not have enough mass to go supernova. A nova event can happen many times. This whole process happens because a white dwarf gets a lot of material from a nearby companion. This material gets heated up and eventually there can be a small explosion.
Sometimes stars form a lot larger than our own Sun and can take a different path. Since they are so large, they can burn and fuse heavy elements which just fuels their demise. They become so dense and hot that even physics can’t take anymore and these stars go supernova. This results in tremendous explosions.
Astronomers once thought that we should be seeing a supernova every hundred years or so in our own galaxy. We are quite far behind. We do not know why there have not been any sightings recently. Everyone is on the lookout though. If we see one soon, it will be an exciting day because these astronomical events are one of a kind!
Matter in Interstellar Space
Emission Nebulae
Dust Clouds
21-Centimeter Radiation
Conclusion
Black Holes
These ultra dense stars come from a supernova. This type of supernova leaves part of the core behind. The reason why is that it is less of an explosion. The solid core does not get blasted into space. Instead, the core now goes through more interesting processes of its own. This core is called a stellar remnant. After everything gets blown away by the shock-wave, all that is left is neutrons. This is why it is called a neutron star and it is a fascinating part of astronomy essentials.
Characteristics of Neutron Stars
A neutron star forms after its core collapses. This process occurs when a star that is approximately 6 times as massive as our Sun explodes in a violent supernova. When this happens, the protons and electrons meld into neutrons. Needless to say, these objects are extremely tiny and dense compared to their previous life as a main star. They are tiny objects with mass greater than our Sun. Think about that. This density we are talking about is the main reason for the strange properties and actions of a neutron star. It seems to break all the rules. For example, the gravity of a neutron star is beyond comprehension. The actual size is comparable to a small county. Hopefully that will give you an idea of how dense it is.
Another property of a neutron star is that it spins! This phenomenon introduces all kinds of wild behavior. Scientists are still trying to learn all about this. So not only does it spin, but it does so quite rapidly. It can spin hundreds of times a second. When this happens, its name is a pulsar. Usually a binary system is responsible for a pulsar. One star gives a lot of its material to the other. These are fascinating objects. They will pulsate at many time intervals. The pulses reach us across astronomical distances but do so more regularly than atomic clocks. They are predicted to do it for millions of years.
These pulsars send matter outward at close to the speed of light. An immense magnetic field combined with rapid rotation sends particles and radiation out quickly in a discrete direction. A neutron star can give out these regular pulses of radiation and the type of depends on how it was formed and other variables. The radiation that we can sometimes detect is in the form of radio waves most often. However, other forms of radiation have also been detected. The periods of most pulsars are very fast. They can pulsate many times a second. Telescopes are used to detect the pulsating flashes.
The last main property is its strong magnetic field. It is also much stronger than its parent magnetic field. The reason is the same too. Decreasing in size so much makes the field much stronger. The average neutron star has a magnetic field around a trillion times stronger. Then there is a type of neutron star that has an even stronger magnetic field. It is the magnetar. These have ferocious magnetic fields. There is nothing in the known universe that has a stronger field.
Fast Pulsars
Regular pulsars have rotational periods of several times a second on average. However, there are a few strange species that can actually rotate several hundred times a second. These almost defy description. If you need something to study one day, these astronomical objects would be worth your time. Most are found in globular clusters. Globular clusters are very old, some of the oldest regions in the universe. They are a constant star forming region. That is probably why neutron stars became pulsars.
So fast pulsars probably formed through interactions with other stars. It could have been a companion or some other object nearby. Whichever it was, the interaction increased the spin rate of the neutron star. That is the best guess as to how this process happens.
Companion Stars
Since a lot of stars have companions, neutron stars are no exception. These are called binary systems. This allows their mass to be determined to a fair degree of accuracy. Astronomers have noticed that many neutron stars are of similar size. That size is slightly larger than our own Sun. This is called the Chandrasekhar limit. This was an incredible discovery. It meant Astronomers needed to find out why this happened.
Binary systems of neutron stars will also emit x-rays. This process is not too well understood, but it is thought to be because gas is pulled away from the companion star. This gas becomes part of an accretion disk which gets super-heated. When it gets hot enough, it starts emitting x-rays. These x-rays have incredible energy associated with them.
Binary Collisions
The two stars can sometimes have a neutron star collision. This is a strange event that releases enormous amounts of energy. It happens when they orbit each other and somehow get too close. This is the beginning of the end. This event is the main source of heavy elements in the universe. These include the valuable gold and platinum.
Gamma Rays
So, gamma rays probably did not create the Incredible Hulk, but how cool would that be if they could! Anyway, I got distracted there, gamma rays are highly energetic blasts of radiation. These rays are very rare too. Astronomers think they come from outside the galaxy. In other words, they come from very far away! There does not seem to be any pattern to them either. Appearances are completely random. That is why they are popular to study.
Their structure seems more akin to a laser beam. Highly concentrated and fast traveling. Astronomers do not know much more than that. Scientists have developed a few different models to explain their behavior. None of which has become universal. One of the problems is they violate our known physics laws. That is indication that our own physics is not substantial enough yet. Scientists have a lot of work to do still.
Black Holes
Black holes are confusing objects. Hollywood and tv does not help with this matter either. The most simple explanation I can offer is they are stellar remnants that have been compressed so much they do not follow our rules of physics anymore. Black holes are not even holes. They are just a super dense neutron star. This neutron star is so dense that it alters our understanding of gravity and density. We can guess and speculate, but scientists do not know anymore than that. If you have read scientific literature for any length of time, then you know they like to speculate and offer what-if scenarios. This is all because no one really understands them. Their entire existence is just a theory at this time. So don’t believe what you see in the movies, no matter how entertaining.
One of the ideas scientists think they are certain about is that a black hole or neutron star can not be above 3 solar masses. That is relative to our Sun. No one is sure why this is the case either. It is thought to be because of the properties of neutrons.
The really difficult thing about black holes is they give no information about themselves. If they exist, they would not give off any kind of radiation. This means no visible light, x-rays, or pulses of any kind. This is because gravity would keep any radiation from leaving. That includes light. That is how powerful gravity is. The only effect we would see is gravitational effects on other objects. So that would be our only clue.
Galaxies
Ours and other galaxies are made up of stars, planets, dust, gas and quite possibly a black hole for the most part. There are reportedly billion of other galaxies just like ours. Ours is fairly typical though, from what we can tell. Astronomers are still studying
what our overall shape is.
When we look at the night sky, we often see an extra bright band sweeping across
the sky. This is made up of a multitude of stars that are very far away. They
are so concentrated the band appears as its own light source in the sky. It's
truly incredible.
Structure of Galaxies
Galaxies can be several different shapes. In fact, the shape can be an indicator
of how that galaxy was formed. This in turn will reveal many of the processes
that happened during the formation.
Stars within any particular galaxy will usually be pretty far apart. They will
also not move far from the galactic plane until they get older. Older stars will
interact with other objects to a greater degree and start to wander slightly.
There is a lot of dust that impedes our view. Because of this, radio astronomy
has been a key factor in discovering the shape of our own and other galaxies.
Our galaxy appears to be football shaped. I remember when that fact came out
that scientists were surprised as they expected it to be another shape.
The Milky Way Sky
On a clear and dark night, you can see a long cloudy band of stars. This is a
portion of our Milky Way galaxy. Why does it appear as a cloudy band of light?
This is a common question. It is because we are inside one of the spiral arms
of our galaxy. That band is us looking out from one of the arms. The spiral arms
appear patchy because of numerous gas clouds that cloud our vision.
Variable Stars
There fast became a need to measure everything around us. We did not know how
many stars were around us, how far away they were, or even what they were made
of. Astronomers even made some pretty bad guesses too. Finally, we discovered
variable stars. Many galaxies seem to have them and they helped Astronomers
greatly.
Variable stars are helpful because their brightness changes as a function of
time. With this information we can infer a great deal. That is why they are
helpful. The Cepheid variables are probably the most famous.
Mapping Our Galaxy With Variables
A cepheid is a pulsating variable star. The pulses happen because they are in a
later stage of life. The pulses are the stars contractions as they try to feed
off hydrogen. These pulses happen on a regular basis. Using this regularity, we
can tell the distance to them and relate to other astronomical objects. The
relationship between their periods and luminosities lets us derive the distance
to them. Since this is so useful, there is an ongoing search for pulsating
variable stars. Finding them lets us map out our galaxy. This is incredibly
useful and fun.
Motion of the Stars
The stars move and they do so constantly. This may be surprising to some since we
also know our planet moves in a constant orbit. In fact, our entire galactic
disk is rotating. The galactic disk includes all the stars, dust, and gas that
it contains.
Motion, of course, depends on how close you are to the galactic center. The
closer you are to the center the faster you would be moving. If you are very
far away then you will be moving slower than objects closer to the center.
There is a term that refers to this kind of motion and it is called differential
motion.
The Halo and Disk
Our galaxy consists of a disk and an outer halo of stars. These are different
components. Stars in the main disk have substantially different properties than
stars in the halo. This is due to age and how they were formed. The main disk
has stars of all ages and is tightly packed. The outer halo has very old stars
and is very sparsely populated. This halo is where we can find some globular
clusters also.
Formation of Galaxies
Ours and other galaxies start as a contracting cloud of gas. This is gas that
is becoming more cold and dense over time. This is what causes the contraction.
The gas was in big globs. There was no overall shape yet because the motion
between everything was pretty random at this point. this is the beginning of how stars form.
As objects started interacting with each other, rotational motion started to
occur. This motion eventually flattened the gas and dust into a relatively
stable disk.
Over time, all the matter in the disk cooled even further. This is when star
formation ceased in our galaxy. Matter collected further into the plane of the
disk. There was a lot of heat loss and motion became less random.
Mass of a Galaxy
The mass of a galaxy is difficult to calculate accurately. However, we can get
decent estimates by studying the motion of objects that are inside that
particular galaxy.
massofgalaxy=size3period2
This is a real rough estimate, but it is a start. As can be seen, galaxies are
beyond large. This is why they exert such gravitational pull on objects close
to them.
Dark Matter
There has been lots of talk about dark matter in the last several years.
Journals have frequent and regular articles that discuss it. Most people are
just confused by it. At its face value, it does not make a lot of sense. I have
read it as being described as invisible. Others have said that we are walking
in a soup of it but we can't feel or see it. There have been many theories!
Another theory is that dark matter is all the hidden subatomic particles we
haven't discovered yet. This is at least interesting but I'm doubtful of it too.
The reason I am doubtful is that right now we don't even have a reason to
suspect there are that many particles floating around freely that violate the
laws of physics and go undetectable. Anyway, that is what I'm thinking, we will
see how things turn out.
Conclusion
So that leads us to the question: what is dark matter? I don't know any more
than the rest of the crackpots out there, including myself in that group, but I
am guessing it is just regular matter. This matter is probably very small and
there are probably dust clouds impeding view and blocking reflections from
light. Since this matter is also in deep space, it is very cold which is why we
can't detect radiation either. That is just my guess so take it with a grain of
salt. Everyone has a different guess. Some make a lot of sense such as brown
dwarfs and some are a bit more outlandish. Hopefully, we will find out more in
the coming years.
Classification of Galaxies
Galaxies are the foundations of the universe and we have identified millions
of them. In this chapter I want to mention and talk about the most defining
features.
Spiral Galaxies
A spiral galaxy has a flattened disk and multiple spiral arms. Our galaxy is a good example. There are more stars in the center of this type of galaxy. Its density is greater there. The way we classify galaxies originates with Edwin Hubble, who made many great discoveries in astronomy. He had a naming convention that used small and large letters. The letters indicated bulges and shapes for example. There were more letters used as well.
Bulges of these galaxies can contain old stars. These older stars do not
contribute as much light as newer stars.
The galactic planes of most galaxies contain large amounts of dust and gas.
Thick areas of gas and dust block our attempts at discovering what is going on.
This is a good example of astronomers having to use radio astronomy to "see"
into them. Visibility is too poor for telescopes to penetrate.
When studying galaxies, it is important to remember that astronomers will not have perfect views. They are inferring a lot from various data. It is pretty accurate though considering we can't actually see it with our eyes.
Elliptical Galaxies
Elliptical galaxies also have a dense nucleus of stars. They do not have spiral arms. There is also not a galactic disk. All these characteristics make ellipticals very different than spiral galaxies.
There is great variety in size and shape among ellipticals. Some are very large! Their shape can vary a lot. A few types are almost circular and others are extremely elliptical. There are many differences in these types of galaxies. This means they formed under widely varying conditions.
There are a few things in common among elliptical galaxies. One of these is very little cold gas. You would expect a galaxy to have massive quantities of it. Instead, there are large quantities of hot gas. There is also very little star formation going on. The large quantities of hot gas would be more plausible if there was a lot of new stars being born. So this is very unusual.
Everything Else
There are countless exceptions to the above categories. I can understand why this may be confusing. It is confusing to me also. Why have categories for a few types but have nothing for a million others? I guess it was just the best that could be done at the time. Remember, you can't actually see these far away galaxies to any detail with a telescope currently.
These exceptions can have all different shapes, sizes, and characteristics. They tend to be rich in resources and have many young stars. This means star formation is still going strong in those galaxies.
They are usually smaller than spiral or ellipticals. This means they contain fewer stars and have less volume in space.
Differences Between Galaxies
So far, we have covered a few different types of galaxies and their main attributes. This includes our own galaxy. So are we like the other galaxies as a whole or are we different? Well, while not entirely unique, we are not really very common either.
Spiral galaxies have several spiral arms. These arms come from the type of star formation that they underwent. Our galaxy and other spirals have large amounts
of cool gas throughout.
Elliptical galaxies are very similar in those characteristics too. Minus the spiral arms, they are very similar to spiral galaxies. Large amounts of cool gas and a dense nucleus are both common attributes.
That makes up just a small fraction of galaxies though. Everything else is different. There are varying degrees of differences but the average galaxy that is not nearby is just wildly different.
Determining Distances
With galaxies further away, better methods had to be discovered to calculate distances. At first we used plain geometry. Then the great cepheid discoveries really helped. Now, we use objects deep in space that we know about. A star, nebulae, or another galaxy that is always the same really helps. We can calculate its brightness based on its distance. That makes it easier to compare to other stars or galaxies.
Groups Of Galaxies
Everything in our universe is in groups or clusters. The atomic nucleus, organisms, planets around our sun, stars around the black whole, and then clusters of galaxies. It is just the way it is. Most matter in the universe is in clumps of similar types of matter.
Galaxies are certainly no exception. They group together because of gravity and other forces. Small numbers are usually called groups. Large numbers of galaxies are referred to as clusters.
Retreat!
Galaxies move along with everything else in the universe. Most are moving away from us and from each other too. This is called dispersion. We know this because astronomers analyze the light of everything they see in space. This is spectrum analysis and it allows us to see the different wavelengths of the light that is being broadcast by any particular object. When the light is reddish it is known as being red-shifted. Red-shifted light occurs when an object is moving away from us.
This is the universal expansion of the universe that you will hear about sometimes. Everything is moving farther apart. To what and to where, we do not know. We just know that it is all moving somewhere.
Active Galaxies
We first tried to classify galaxies according to their shape. This worked out fair but not great. We can discuss galaxies with respect to their energy too though. Our home galaxy is a normal one. It is pretty average as far as size and brightness. There are other types of galaxies though. Active galaxies are far more energetic. Because of this, they are of great interest to astronomers.
The funny thing is that we can't really tell by looking at them in optical wavelengths. They start to get exciting when seen at wavelengths above and below optical frequencies.
Among active galaxies, there are still more subtypes. All of these are highly energetic galaxies. There are not many of these. It is rare to find a new one.
Radio Galaxies
These active galaxies spew out a lot of energy. They do so in mostly the radio wavelengths of the spectrum. They look a lot different than our own normal galaxy. Size is another difference that we share. Radio galaxies are much larger. The energy emitted can be several times larger than our own galaxy. That makes the energy enormous. Radio galaxies will usually have visible jets and lobes.
The jets are where matter has been violently ejected perpendicular to the core. Matter that is ejected like this can be a long way from its parent galaxy. Lobes are areas of radio emissions that can be extremely large.
Galactic Energy
The energy production in active galaxies is astounding. This is what is meant by saying a galaxy is energetic. There are a few reasons for this. One of the main ones is that the central black hole is thought to be much larger. It is so large because more material falls into it. More material means more friction and release of energy in forms of radiation.
Conclusion
Accretion disks power the displays of energy we know as jets. Matter drawn in increases the disk's mass. Material that leaves causes friction in the disk and more energy is released and used to power this process to an even greater degree. It's simply amazing! Jets can appear far away in most cases. These jets are made up of electrons and protons that are blasted out into space perpendicular from the disk.
Dark Matter
While measuring our own galaxy, we can see there must be a large amount of unknown matter here. We can’t see it or detect it. That is why it is such a big mystery. We first noticed this discrepancy because objects were not following the gravitational laws in the ways they should. So, dark matter is stuff that we can not see and gives off no detectable energy. Seems weird, for sure, but that is the consensus right now.
Expansion Of The Universe
Since we discovered the universe was expanding, there have been constant theories about why. Astronomers have also theorized how long the expansion would last. There is still no consensus on anything. Scientists have long thought that gravity would cause the expansion to stop. Intuitively, this would be the normal thought of most people. After all, gravity is strong and affects cosmic objects from vast distances away.
Thanks to the Hubble telescope, we know the expansion velocity of the universe has increased. This makes no sense at all. Something must cause the acceleration, obviously.
Scientists love a chance to name something new, so we now have dark energy. This dark energy, which is just a vague and generic placeholder, is our cause of universal acceleration.
Early physicists thought dark energy was just a property of space itself. What looks empty to you and me is not empty at all. It is full of energy and potential. In fact, Einstein thought that space could propagate more space. This could certainly be a hypothesis to expand the universe.
So, these are all the reasons that the observable mass of galaxies is way off. The mass is not just way off; it is magnitudes different than it should be.
Mass Of Galaxies
Estimating the masses of galaxies is hard. They are far away and we can only see faint details. So, we have to use indirect methods. These indirect methods are educated guesses based on their characteristics. The main such method is using the length of the galaxy arms versus distance from their galactic center. Then the velocities of these moving arms are calculated, which gives us a good estimate of mass.
These calculations are all done using Newton’s Laws. So far, these methods work on our own galaxy and others close by. They give us a range of mass to go by, which helps us infer that a lot of mass is missing. This is what astronomers have named dark matter.
For galaxies farther away, we have to use more indirect methods. This usually involves analyzing spectroscopic lines. When the lines change their width, this shows rotation speed. Of course, once we have that, then we can guess size and mass.
We have analyzed many galaxies in this way. In doing so, astronomers have realized that certain types of galaxies often come within a particular range of masses. This is great because, as we compile more information, we can make even better, more accurate guesses at these far away objects.
Matter We Can See
Astronomers think that most galaxies contain large amounts of dark matter. Spiral and elliptical galaxies have similar amounts of missing matter. So, the amount of dark matter may have nothing to do with the type of galaxy. All galaxies seem to have dark matter.
In fact, the accepted figure is around 27% dark matter per galaxy. I can’t say how they get a figure that precise. It’s done by people smarter than I. Regardless, that leaves inconsiderable matter that we can actually observe.
Conclusion
In this document, I tried to discuss the reason we are missing something. We are missing the cause of galaxies having much less mass than they should and why the universe is expanding faster and faster. These events are related, I feel. There is something big we are all missing. It just has not been discovered yet.